Making drones suitable for cities
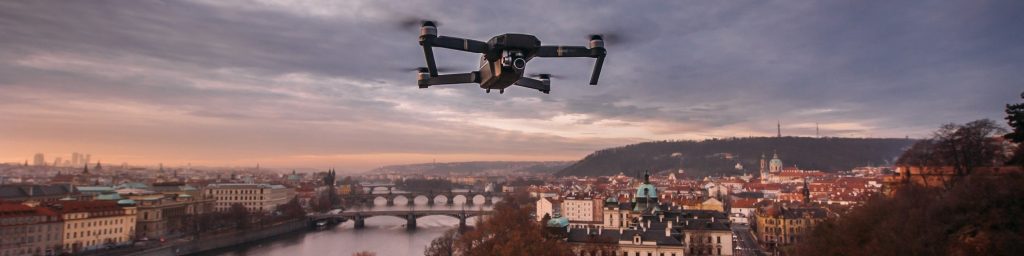
With technology for drones far advanced, the next step is to ensure they can fly safely in cities. Image credit: CC0 via Unsplash
The Spanish resort town of Benidorm is known for its sandy beaches with clear waters, a skyline dominated by towering hotels and tourists from northern Europe. But one day in February, it also served as a testing ground for European society’s future with drones.
Since the local economy depends on tourism during the summer, Benidorm is relatively empty in winter – and that’s a plus when it comes to safety while testing unmanned aerial vehicles (UAVs). The tall buildings that dominate the skyline also stand in nicely for those of a big city.
Sun, sea and…satellite signals
In sum, it’s an ideal place to try out new drone technology. And an EU-funded project called DELOREAN has done just that – testing new types of satellite tracking for drones on 9 February.
‘Benidorm’s skyline is quite similar to what you would find in larger cities like, say, New York,’ said Santiago Soley, the project coordinator who is also chief executive officer of Spanish aeronautics-engineering company Pildo Labs. ‘Generally, regulations limit drone flights over dense urban areas. It’s the first time in Europe we did these intense tests in a challenging city environment.’
Drones have been a hyped technology for years, during which the media popularised predictions that such aircraft would soon be used for all kinds of daily services including delivering packages to people’s doorsteps. Yet so far, widespread civilian use has failed to take off.
The bottleneck is safety and the need to demonstrate to city governments that drones can be operated in large numbers in populated areas without being a hazard. If a UAV crashes onto a busy street or into a plane that’s landing or taking off, the result could be severe damage or even deaths.
“Drone technology is getting there.”
– Santiago Soley, DELOREAN
Scientists and companies are now addressing these concerns – and the experiments in Benidorm might hold the key to the future success of drones.
‘Drone technology is getting there – it’s the least of our problems,’ said Soley. ‘What’s more important is to demonstrate how drones would safely be deployed over cities.’
DELOREAN is wrapping up after three years. The main goal was to develop navigation and positioning requirements for urban air services and show how the European Global Navigation Satellite System, or EGNSS, can help.
Non-GPS options
Drones need to know exactly where they are at all times. For that, UAVs currently rely on satellites, mostly the US Global Positioning System, or GPS. Another alternative to GPS is Europe’s Galileo network.
DELOREAN is also testing Galileo’s potential for drones.
While led by Pildo Labs, the project has featured an international consortium whose members include France-based aircraft manufacturer Airbus, Spanish postal-servicer provider Correos and the European Organisation for the Safety of Air Navigation, or Eurocontrol, in Belgium.
A challenge for satellite tracking in urban areas is that signals might be deflected or otherwise hindered by buildings. Galileo will help avoid such disruptions because of the waveform and structure of its signals, according to Soley.
In addition, Galileo is pioneering new services that could pinpoint drones’ locations with higher accuracy – something DELOREAN tested in Benidorm.
Furthermore, Galileo adds a layer of security. An authentication service that allows the drone to verify whether the satellite signal is real would counter any future efforts by criminal groups to misdirect UAVs and steal their contents through fake signals, according to Soley.
Airborne parcel deliveries
If experiments of the kinds conducted by DELOREAN prove successful, many applications could open up.
“Before businesses like urban air delivery can develop, we first need safety.”
– Professor Luis Moreno Lorente, LABYRINTH
While drones are already in use over cities, it is often in small-scale operations by local authorities. Police departments, for one, use them to monitor crowds or track speeding cars.
‘There are limitations on drone flights and you need to close the area,’ said Soley. ‘At the technical level, however, the flights are quite easy to handle.’
The next step could be mass urban air delivery. No more vans zigzagging through city streets with all the congestion and pollution.
Instead, fleets of drones would drop off packages across town. Companies like Amazon are already rolling out these services in limited areas.
‘Logistics will, I think, be one of the most promising uses of drones,’ said Soley.
Self-flying craft
An EU-funded project called LABYRINTH is tackling the challenge of ensuring that autonomous drones keep track of each other.
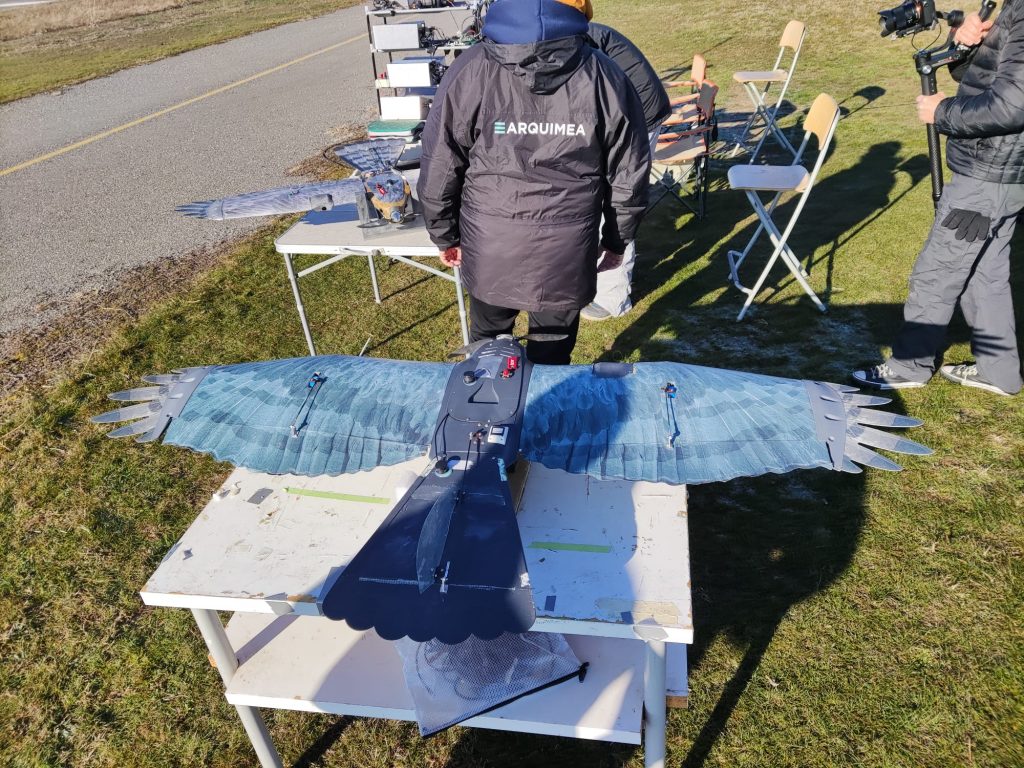
An ARQUIMEA drone being tested in Marugán, Segovia, Spain. © Labyrinth, 2023
Autonomous drones require no ground-based human pilots, who are generally needed for the current generation of UAVs.
‘In the future, those drones will be operated autonomously – they will fly themselves,’ said Luis Moreno Lorente, the project coordinator and a professor of systems engineering and automation at the University Carlos III of Madrid in Spain. ‘But if you want to do that safely, you need to know exactly where each one of them is located.’
LABYRINTH, which is due to end in May after three years, is developing software that acts as an air traffic control system for drones. The 3D position of each is tracked and the aircraft then relays this information to other drones in the vicinity so they don’t crash into each other.
Similarly, if a drone faces technical troubles – say one of its motors fails – it needs to be able to direct other UAVs away from it.
‘Before businesses like urban air delivery can develop, we first need safety,’ said Moreno Lorente. ‘That’s what we’re building now.’
Together, LABYRINTH and DELOREAN are helping to clear the way for a future in which large numbers of drones fly over cities.
‘It’s just a matter of time before they do,’ said Moreno Lorente.
Watch the video
This article was originally published in Horizon, the EU Research and Innovation magazine.
Is China out to spy on us through drones and other tech? Perhaps that’s not the question we should be asking
Researchers use novel approach to teach robot to navigate over obstacles
Soft e-skin that communicates with the brain
Robot Talk Episode 49 – Nick Hawes
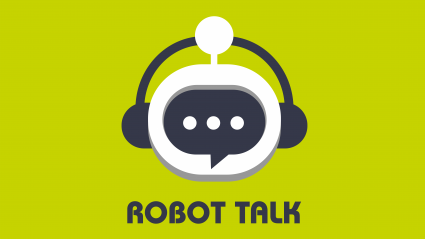
Claire chatted to Nick Hawes from the University of Oxford all about robot decision-making, long-term autonomy, and artificial intelligence.
Nick Hawes is a Professor of AI and Robotics at the University of Oxford, where he directs the Oxford Robotics Institute (ORI). Within the ORI he leads the Goal-Oriented Autonomous Long-Lived Systems (GOALS) group which researches decision-making for autonomous systems under uncertainty, including robots and human-robot teams. Recent highlights include the deployment of an autonomous mission planning stack on a quadruped at an active nuclear site, and on an autonomous underwater vehicle harvesting data from a sensor network.
OrionStar Robotics to Unveil Fully-Automated Restaurant Solution at NRA 2023
DreamWaQer: A quadrupedal robot that can walk in the dark
How to Reduce Chatter in Robotic Milling Applications
Additively manufacturing soft robots could reduce waste, increase performance
Got robot delivery? New research demonstrates need for robot-friendly infrastructure
MiniMACS6 Stabilizes the Furuta Pendulum
Jellyfish-like robots could one day clean up the world’s oceans
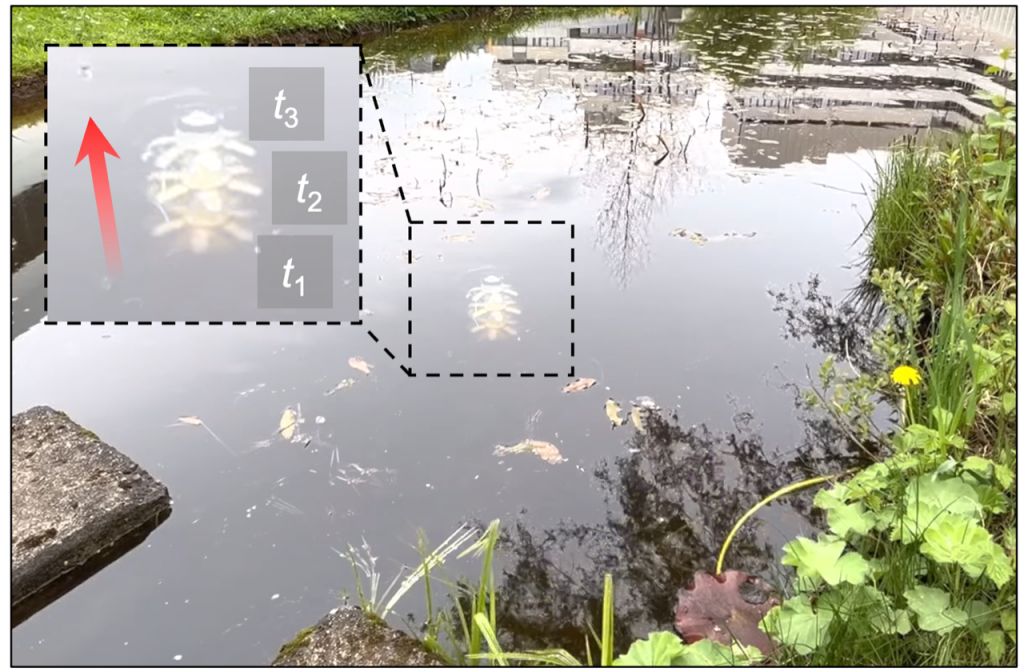
Most of the world is covered in oceans, which are unfortunately highly polluted. One of the strategies to combat the mounds of waste found in these very sensitive ecosystems – especially around coral reefs – is to employ robots to master the cleanup. However, existing underwater robots are mostly bulky with rigid bodies, unable to explore and sample in complex and unstructured environments, and are noisy due to electrical motors or hydraulic pumps. For a more suitable design, scientists at the Max Planck Institute for Intelligent Systems (MPI-IS) in Stuttgart looked to nature for inspiration. They configured a jellyfish-inspired, versatile, energy-efficient and nearly noise-free robot the size of a hand. Jellyfish-Bot is a collaboration between the Physical Intelligence and Robotic Materials departments at MPI-IS. “A Versatile Jellyfish-like Robotic Platform for Effective Underwater Propulsion and Manipulation” was published in Science Advances.
To build the robot, the team used electrohydraulic actuators through which electricity flows. The actuators serve as artificial muscles which power the robot. Surrounding these muscles are air cushions as well as soft and rigid components which stabilize the robot and make it waterproof. This way, the high voltage running through the actuators cannot contact the surrounding water. A power supply periodically provides electricity through thin wires, causing the muscles to contract and expand. This allows the robot to swim gracefully and to create swirls underneath its body.
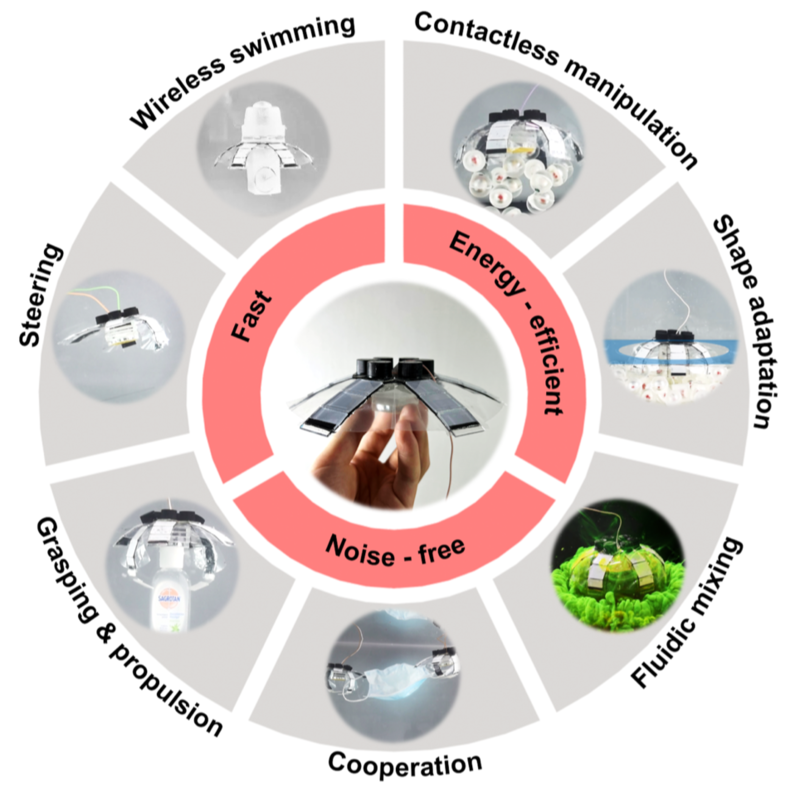
“When a jellyfish swims upwards, it can trap objects along its path as it creates currents around its body. In this way, it can also collect nutrients. Our robot, too, circulates the water around it. This function is useful in collecting objects such as waste particles. It can then transport the litter to the surface, where it can later be recycled. It is also able to collect fragile biological samples such as fish eggs. Meanwhile, there is no negative impact on the surrounding environment. The interaction with aquatic species is gentle and nearly noise-free”, Tianlu Wang explains. He is a postdoc in the Physical Intelligence Department at MPI-IS and first author of the publication.
His co-author Hyeong-Joon Joo from the Robotic Materials Department continues: “70% of marine litter is estimated to sink to the seabed. Plastics make up more than 60% of this litter, taking hundreds of years to degrade. Therefore, we saw an urgent need to develop a robot to manipulate objects such as litter and transport it upwards. We hope that underwater robots could one day assist in cleaning up our oceans.”
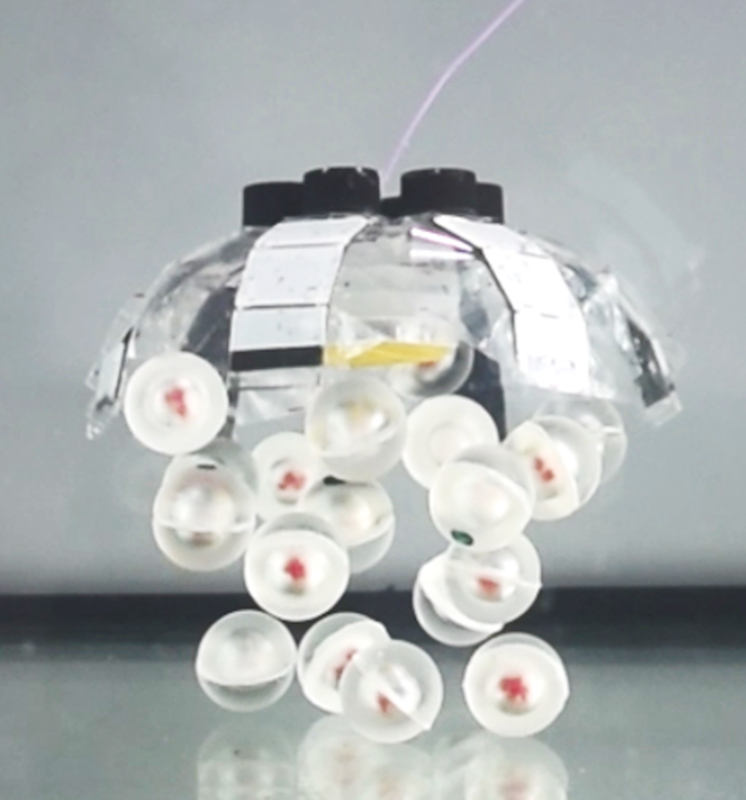
Jellyfish-Bots are capable of moving and trapping objects without physical contact, operating either alone or with several in combination. Each robot works faster than other comparable inventions, reaching a speed of up to 6.1 cm/s. Moreover, Jellyfish-Bot only requires a low input power of around 100 mW. And it is safe for humans and fish should the polymer material insulating the robot one day be torn apart. Meanwhile, the noise from the robot cannot be distinguished from background levels. In this way Jellyfish-Bot interacts gently with its environment without disturbing it – much like its natural counterpart.
The robot consists of several layers: some stiffen the robot, others serve to keep it afloat or insulate it. A further polymer layer functions as a floating skin. Electrically powered artificial muscles known as HASELs are embedded into the middle of the different layers. HASELs are liquid dielectric-filled plastic pouches that are partially covered by electrodes. Applying a high voltage across an electrode charges it positively, while surrounding water is charged negatively. This generates a force between positively-charged electrode and negatively-charged water that pushes the oil inside the pouches back and forth, causing the pouches to contract and relax – resembling a real muscle. HASELs can sustain the high electrical stresses generated by the charged electrodes and are protected against water by an insulating layer. This is important, as HASEL muscles were never before used to build an underwater robot.
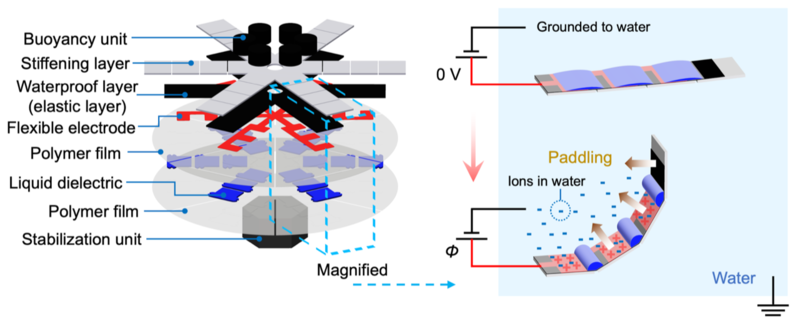
The first step was to develop Jellyfish-Bot with one electrode with six fingers or arms. In the second step, the team divided the single electrode into separated groups to independently actuate them.
“We achieved grasping objects by making four of the arms function as a propeller, and the other two as a gripper. Or we actuated only a subset of the arms, in order to steer the robot in different directions. We also looked into how we can operate a collective of several robots. For instance, we took two robots and let them pick up a mask, which is very difficult for a single robot alone. Two robots can also cooperate in carrying heavy loads. However, at this point, our Jellyfish-Bot needs a wire. This is a drawback if we really want to use it one day in the ocean”, Hyeong-Joon Joo says.
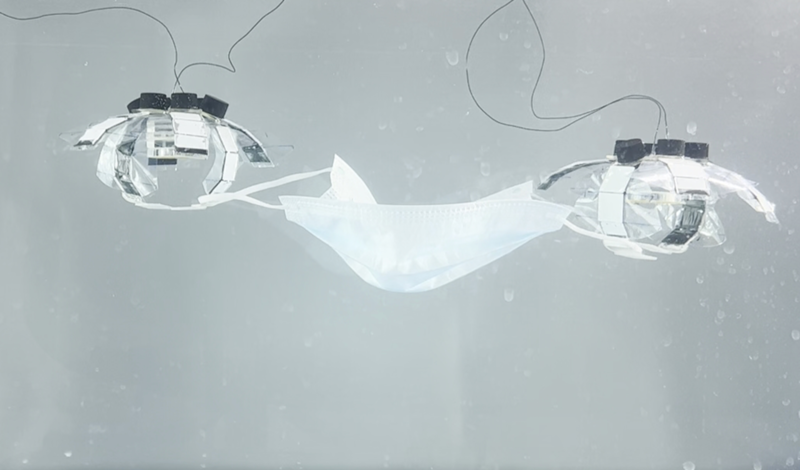
Perhaps wires powering robots will soon be a thing of the past. “We aim to develop wireless robots. Luckily, we have achieved the first step towards this goal. We have incorporated all the functional modules like the battery and wireless communication parts so as to enable future wireless manipulation”, Tianlu Wang continues. The team attached a buoyancy unit at the top of the robot and a battery and microcontroller to the bottom. They then took their invention for a swim in the pond of the Max Planck Stuttgart campus, and could successfully steer it along. So far, however, they could not direct the wireless robot to change course and swim the other way.
Knowing the team, it won’t take long to achieve this goal.