The Growth of Non-Industrial Robotics Is Driving a Diversification of New Robotics Applications, According to Tractica
Why AI robot toys could be good for kids
Growing bio-inspired shapes with a 300-robot swarm
Work by I. Slavkov, D. Carrillo-Zapata, N. Carranza, X. Diego, F. Jansson, J. Kaandorp, S. Hauert, J. Sharpe
Our work published today in Science Robotics describes how we grow fully self-organised shapes using a swarm of 300 coin-sized robots. The work was led by James Sharpe at EMBL and the Centre for Genomic Regulation (CRG) in Barcelona – together with my team at the Bristol Robotics Laboratory and University of Bristol.
Here’s a video summarising the results, or you can read the paper here:
Self-organised shapes
Nature is capable of producing impressive functional shapes throughout embryonic development. Broadly, there are two ways to form these shapes.
1) Top-down control. Cells have access to information about their position through some coordinate system, for example generated through their molecular gradients. Cells use this information to decide their fate, which ultimately creates the shapes. There are beautiful examples of this strategy being used for robot swarms, check here for work by Rubenstein et al. (video).
2) Local self-organisation. Cells generate reaction-diffusion systems, such as those described by Alan Turing, resulting in simple periodic patterns. Cells can use these patterns to decide their fate and the resulting shape.
We use the second strategy, here’s how it works.
Patterning
We start from a swarm of 300 closely packed robots in a disc – each running the same code. Each robot stores two morphogens u and v, which you can think of as virtual chemical signals. Morphogen u activates itself and the other morphogen v, whereas v inhibits itself and the other morphogen u – this is a ‘reaction’ network. ‘Diffusion’ of u and v happens through communication from robot to robot. Symmetry breaking caused by the ‘reaction-diffusion’ system results in spots emerging on the swarm (or stripes if we change the parameters!). Areas with high-levels of morphogens are shown in green – that’s what we call a “Turing spot”.
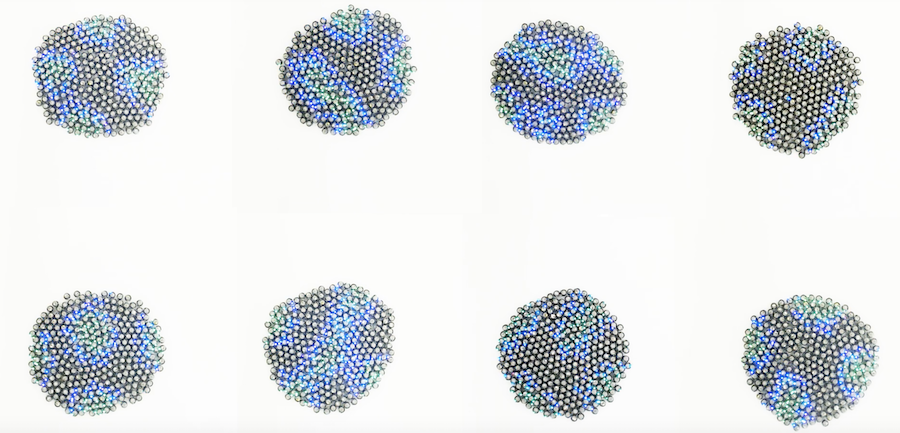
Tissue movement
In biology, cells may die or multiply depending on their patterning. As we can’t do either of those things with robots, we simply move robots from areas where they are no longer needed to areas of growth. The general idea is that robots that are on the edge of the swarm, and are not in a Turing spot, move along the edge of the swarm until they are near the spot. This causes protrusions to grow at the location of the Turing spots.

Following these simple rules, we are able to grow shapes in a repeatable manner, although all the shapes are slightly different. If you watch the video, you’ll see that these shapes look quite organic. We did over 20 experiments with large robot swarms, each one taking about 3 hours.
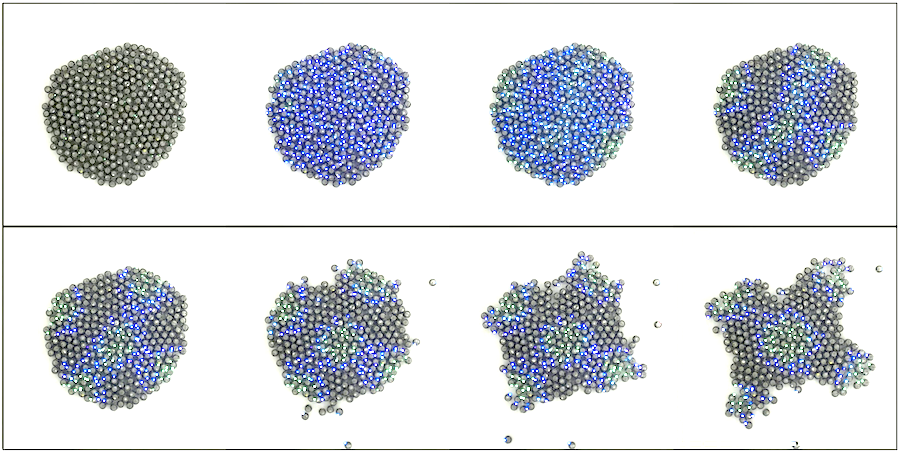
Because the rules are so simple, and only rely on local information, we get adaptability and robustness for free.
Adaptability
First, as the shape grows, the Turing spots move, showing that the patterning adapts to the shape of the swarm, and that the shape further adapts to the patterning. Second, we can easily change the starting configuration of the swarm (smaller number of robots, or a ‘rectangular’ starting conditions) and the shape still forms.
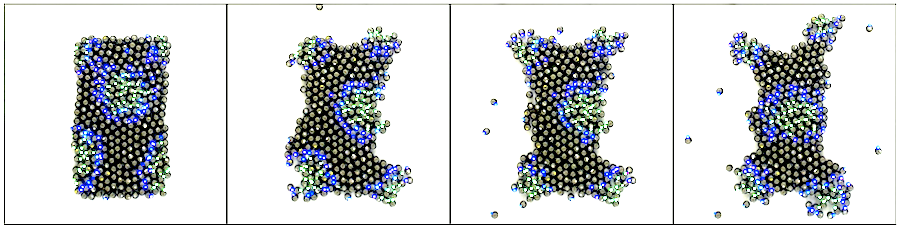
Robustness
Chopping off a protrusion, causes the robots to regrow it, or to reallocate robots to other protrusions in the swarm. Splitting the swarm causes it to self-heal.
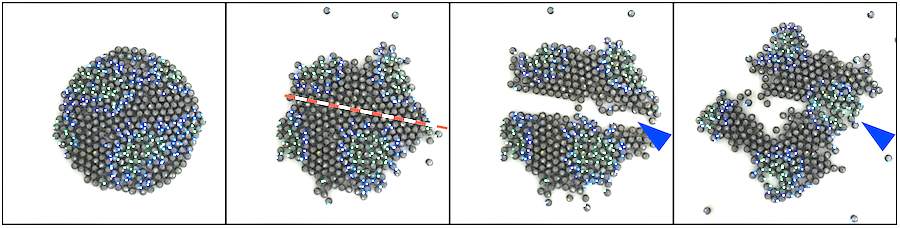
Potential for real world applications
While inspiration was taken from nature to grow the swarm shapes, the goal is ultimately to make large robot swarms for real-world applications. Imagine hundreds or thousands of tiny biodegradable robots growing shapes to explore a disaster environment after an earthquake or fire, or sculpting themselves into a dynamic 3D structure such as a temporary bridge that could automatically adjust its size and shape to fit any building or terrain. There is still a long way to go however, before we see such swarms outside the laboratory.
Team
James Sharpe (EMBL Barcelona) led the Swarm-Organ project, which was initiated at the Centre for Genomic Regulation (CRG) when Sharpe was a group leader there. Sabine Hauert (Bristol Robotics Laboratory and University of Bristol) was the key senior collaborator. Other collaborators were Fredrik Jansson (currently employed at Centrum Wiskunde & Informatica – CWI) and Jaap Kaandorp (University of Amsterdam – UvA).
Paper
You can read more in the paper Slavkov, I., Zapata D. C. et al., Science Robotics (2018).
Funding
The research leading to these results has received funding from the European Union Seventh Framework Programme (FP7) under grant agreement n° 601062, and the EPSRC Centre for Doctoral Training in Future Autonomous and Robotic Systems (FARSCOPE) at the Bristol Robotics Laboratory.
When high tech goes underground
From robotic companions to third thumbs, machines can change the human brain
Robots are being programmed to adapt in real time
It Takes a Swarm: These Robots Talk to Each Other, Make Decisions as a Group
MiR200 Improves Safety, Quality, and Competitiveness at Metro Plastics
Autonomous Mobility Grows Its Sea Legs — Toyota AI Investment in Sea Machines
Call for robot holiday videos 2018
That’s right! You better not run, you better not hide, you better watch out for brand new robot holiday videos on Robohub! Drop your submissions down our chimney at sabine.hauert@robohub.org and share the spirit of the season.
For inspiration, here are our first submissions .
Robot makes world-first baby coral delivery to Great Barrier Reef
In a world first, an undersea robot has dispersed microscopic baby corals (coral larvae) to help scientists working to repopulate parts of the Great Barrier Reef during this year’s mass coral spawning event.
Six weeks after winning the Great Barrier Reef Foundation’s $300,000 Out of the Blue Box Reef Innovation Challenge, Southern Cross University’s Professor Peter Harrison and QUT’s Professor Matthew Dunbabin trialled the ground-breaking initiative on Vlasoff Reef, near Cairns in north Queensland.
Professor Dunbabin engineered QUT’s reef protector RangerBot into LarvalBot specifically for the coral restoration project led by Professor Harrison.
The project builds on Professor Harrison’s successful larval reseeding technique piloted on the southern Great Barrier Reef in 2016 and 2017 in collaboration with the Great Barrier Reef Foundation, the Great Barrier Reef Marine Park Authority (GBRMPA) and Queensland Parks & Wildlife Service (QPWS), following successful small scale trials in the Philippines funded by the Australian Centre for International Agricultural Research.
Watch interview below
“This year represents a big step up for our larval restoration research and the first time we’ve been able to capture coral spawn on a bigger scale using large floating spawn catchers then rearing them into tiny coral larvae in our specially constructed larval pools and settling them on damaged reef areas,” Professor Harrison said.
“Winning the GBRF’s Reef Innovation Challenge meant that we could increase the scale of the work planned for this year using mega-sized spawn catchers and fast track an initial trial of LarvalBot as a novel method of dispersing the coral larvae out on to the Reef.
“With further research and refinement, this technique has enormous potential to operate across large areas of reef and multiple sites in a way that hasn’t previously been possible.
“We’ll be closely monitoring the progress of settled baby corals over coming months and working to refine both the technology and the technique to scale up further in 2019.”
This research and the larval production process was also directly supported by the recent successful SBIR 2018 Coral larval restoration research project on Vlasoff Reef led by Professor Harrison with Katie Chartrand (James Cook University) and Associate Professor David Suggett (University of Technology Sydney), in collaboration with Aroona Boat Charters, the GBRMPA and QPWS.
With a current capacity to carry around 100,000 coral larvae per mission and plans to scale up to millions of larvae, the robot gently releases the larvae onto damaged reef areas allowing it to settle and over time develop into coral polyps or baby corals.
Professor Dunbabin said LarvalBot could be compared to ‘an underwater crop duster’ operating very safely to ensure existing coral wasn’t disturbed.
“During this year’s trial, the robot was tethered so it could be monitored precisely but future missions will see it operate alone and on a much larger scale,” Professor Dunbabin said.
“Using an iPad to program the mission, a signal is sent to deliver the larvae and it is gently pushed out by LarvalBot. It’s like spreading fertiliser on your lawn.
“The robot is very smart, and as it glides along we target where the larvae need to be distributed so new colonies can form and new coral communities can develop.
“We have plans to do this again in Australia and elsewhere and I’m looking forward to working with Professor Harrison and Southern Cross University, the Great Barrier Reef Foundation and other collaborators to help tackle an important problem.”
This project builds on the work by Professor Dunbabin who developed RangerBot to help control the coral-killing crown-of-thorns starfish which is responsible for 40 per cent of the reef’s decline in coral cover.
Great Barrier Reef Foundation Managing Director Anna Marsden said, “It’s exciting to see this project progress from concept to implementation in a matter of weeks, not years. The recent IPCC report highlights that we have a very short window in which to act for the long term future of the Reef, underscoring the importance of seeking every opportunity to give our reefs a fighting chance.
“This project is testament to the power of collaboration between science, business and philanthropy. With the support of the Tiffany & Co. Foundation, whose longstanding support for coral reef conservation globally spans almost two decades, our international call for innovations to help the Reef has uncovered a solution that holds enormous promise for restoring coral reefs at scales never before possible.”
Following the success of this initial trial in 2018, the researchers plan to fully implement their challenge-winning proposal in 2019, building even larger mega spawn-catchers and solar powered floating larval incubation pools designed to rear hundreds of millions of genetically diverse, heat-tolerant coral larvae to be settled on damaged reefs through a combination of larval clouds and LarvalBots.
A new drone can change its shape to fly through a narrow gap
A research team from the University of Zurich and EPFL has developed a new drone that can retract its propeller arms in flight and make itself small to fit through narrow gaps and holes. This is particularly useful when searching for victims of natural disasters.
Inspecting a damaged building after an earthquake or during a fire is exactly the kind of job that human rescuers would like drones to do for them. A flying robot could look for people trapped inside and guide the rescue team towards them. But the drone would often have to enter the building through a crack in a wall, a partially open window, or through bars – something the typi-cal size of a drone does not allow.
To solve this problem, researchers from Scaramuzza lab at the University of Zurich and Floreano lab at EPFL created a new kind of drone. Both groups are part of the National Centre of Competence in Research (NCCR) Robotics funded by the Swiss National Science Foundation. Inspired by birds that fold their wings in mid-air to cross narrow passages, the new drone can squeeze itself to pass through gaps and then go back to its previous shape, all the while continuing to fly. And it can even hold and transport objects along the way.
Mobile arms can fold around the main frame
“Our solution is quite simple from a mechanical point of view, but it is very versatile and very au-tonomous, with onboard perception and control systems,” explains Davide Falanga, researcher at the University of Zurich and the paper’s first author. In comparison to other drones, this morphing drone can maneuver in tight spaces and guarantee a stable flight at all times.
The Zurich and Lausanne teams worked in collaboration and designed a quadrotor with four pro-pellers that rotate independently, mounted on mobile arms that can fold around the main frame thanks to servo-motors. The ace in the hole is a control system that adapts in real time to any new position of the arms, adjusting the thrust of the propellers as the center of gravity shifts.
“The morphing drone can adopt different configurations according to what is needed in the field,” adds Stefano Mintchev, co-author and researcher at EPFL. The standard configuration is X-shaped, with the four arms stretched out and the propellers at the widest possible distance from each other. When faced with a narrow passage, the drone can switch to a “H” shape, with all arms lined up along one axis or to a “O” shape, with all arms folded as close as possible to the body. A “T” shape can be used to bring the onboard camera mounted on the central frame as close as possible to objects that the drone needs to inspect.
First step to fully autonomous rescue searches
In the future, the researchers hope to further improve the drone structure so that it can fold in all three dimensions. Most importantly, they want to develop algorithms that will make the drone truly autonomous, allowing it to look for passages in a real disaster scenario and automatically choose the best way to pass through them. “The final goal is to give the drone a high-level instruction such as ‘enter that building, inspect every room and come back’ and let it figure out by itself how to do it,” says Falanga.
Literature
Davide Falanga, Kevin Kleber, Stefano Mintchev, Dario Floreano, Davide Scaramuzza. The Foldable Drone: A Morphing Quadrotor that can Squeeze and Fly. IEEE Robotics and Auto-mation Letter, 10 December 2018. DOI:10.1109/LRA.2018.2885575
Drones and satellite imaging to make forest protection pay
by Steve Gillman
Every year 7 million hectares of forest are cut down, chipping away at the 485 gigatonnes of carbon dioxide (CO2) stored in trees around the world, but low-cost drones and new satellite imaging could soon protect these carbon stocks and help developing countries get paid for protecting their trees.
‘If you can measure the biomass you can measure the carbon and get a number which has value for a country,’ said Pedro Freire da Silva, a satellite and flight system expert at Deimos Engenharia, a Portuguese technology company.
International financial institutions, such as the World Bank and the European Investment Bank, provide developing countries with economic support to keep forests’ carbon stocks intact through the UN REDD+ programme.
The more carbon a developing country can show it keeps in its forests, the more money the government could get, which would give them a greater incentive to protect these lands. But, according to Silva, these countries often lack the tools to determine the exact amount of carbon stored in their forests and that means they could be missing out on funding.
‘If you have a 10% error in your carbon stock (estimation), that can have a financial value,’ he said, adding that it also takes governments a lot of time and energy to collect the relevant data about their forests.
To address these challenges, a project called COREGAL developed automated low-cost drones that map biomass. They put a special sensor on drones that fly over forests and analyse Global Positioning System (GPS) and Galileo satellite signals as they bounce back through a tree canopy, which then reveals the biomass density of an area and, in turn, the carbon stocks.
‘The more leaves you have, the more power (from GPS and Galileo) is lost,’ said Silva who coordinated the project. This means when the drone picks up weaker satellite navigation readings there is more biomass below.
‘If you combine this data with satellite data we get a more accurate map of biomass than either would (alone),’ he added.
Sentinels
The project trialled their drone prototype in Portugal, with Brazil in mind as the target end user as it is on the frontline of global deforestation. According to Brazilian government data, an area about five times to size of London was destroyed between August 2017 and July this year.
COREGAL’s drones could end up enabling countries such as Brazil to access more from climate funds, in turn creating a stronger incentive for governments to protect their forests. Silva also believes the drones could act as a deterrent against illegal logging.
‘If people causing deforestation know that there are (drone) flight campaigns or people going to the field to monitor forests it can demotivate them,’ he said. ‘It is like a sentinel system.’
In the meantime, governments in other developing countries still need the tools to help them fight deforestation. According to Dr Thomas Häusler, a forest and climate expert at GAF, a German earth observation company, the many drivers of deforestation make it very difficult to sustainably manage forests.
‘(Deforestation) differs between regions and even in regions you have different drivers,’ said Dr Häusler. ‘In some countries they (governments) give concessions for timber logging and companies are (then) going to huge (untouched forest) areas to selectively log the highest value trees.’
Logging like this is occurring in Brazil, central Africa and Southeast Asia. When it happens, Dr Häusler says this can cause huge collateral damage because loggers leave behind roads that local populations use to access previously untouched forests which they further convert for agriculture or harvest wood for energy.
Demand for timber and agricultural produce from developed countries can also drive deforestation in developing countries because their governments see the forest as a source of economic development and then allow expansion.
With such social, political and economic dependency, it can be difficult, and expensive, for governments to implement preventative measures. According to Dr Häusler, to protect untouched forests these governments should be compensated for fighting deforestation.
‘To be compensated you need strong (forest) management and observation (tools),’ said Dr Häusler, who is also the coordinator of EOMonDis, a project developing an Earth-observation-based forest monitoring system that aims to support governments.
Domino effect
They combine high-resolution data from the European Sentinel satellites, available every five days through Copernicus, the EU’s Earth observation system, along with data from the North American Landsat-8 satellite.
Automated processing using special algorithms generates detailed maps on the current and past land use and forest situation to identify the carbon-rich forest areas. The project also has access to satellite data going as far back as the 1970s which can be used to determine how much area has been affected by deforestation.
Like COREGAL, using these maps, and the information they contain, a value is put on the most carbon-rich forest areas, meaning countries can access more money from international financial institutions. The project is almost finished and they soon hope to have a commercially viable system for use.
‘The main focus is the climate change reporting process for countries who want compensation in fighting climate change,’ said Dr Häusler. ‘We can support this process by showing the current land-use situation and show the low and high carbon stocks.’
Another potential user of this system is the international food industry that sells products containing commodities linked to deforestation such as palm oil, cocoa, meat and dairy. In response to their contribution, and social pressure, some of these big companies have committed to zero-deforestation in their supply chain.
‘When someone (a company) is declaring land as zero deforestation, or that palm plantations fit into zero deforestation, they have to prove it,’ said Dr Häusler. ’And a significant result (from the project) is we can now prove that.’
Dr Häusler says the system will help civil society and NGOs who want to make sure industry or governments are behaving themselves as well as allow the different groups to make environmentally sound decisions when choosing land for different purposes.
‘We can show everybody – the government, NGO stakeholders, but also the industry – how to better select the areas they want to use.’
The research in this article was funded by the EU. If you liked this article, please consider sharing it on social media.
3Q: Aleksander Madry on building trustworthy artificial intelligence
Photo courtesy of CSAIL
By Kim Martineau
Machine learning algorithms now underlie much of the software we use, helping to personalize our news feeds and finish our thoughts before we’re done typing. But as artificial intelligence becomes further embedded in daily life, expectations have risen. Before autonomous systems fully gain our confidence, we need to know they are reliable in most situations and can withstand outside interference; in engineering terms, that they are robust. We also need to understand the reasoning behind their decisions; that they are interpretable.
Aleksander Madry, an associate professor of computer science at MIT and a lead faculty member of the Computer Science and Artificial Intelligence Lab (CSAIL)’s Trustworthy AI initiative, compares AI to a sharp knife, a useful but potentially-hazardous tool that society must learn to weild properly. Madry recently spoke at MIT’s Symposium on Robust, Interpretable AI, an event co-sponsored by the MIT Quest for Intelligence and CSAIL, and held Nov. 20 in Singleton Auditorium. The symposium was designed to showcase new MIT work in the area of building guarantees into AI, which has almost become a branch of machine learning in its own right. Six faculty members spoke about their research, 40 students presented posters, and Madry opened the symposium with a talk the aptly titled, “Robustness and Interpretability.” We spoke with Madry, a leader in this emerging field, about some of the key ideas raised during the event.
Q: AI owes much of its recent progress to deep learning, a branch of machine learning that has significantly improved the ability of algorithms to pick out patterns in text, images and sounds, giving us automated assistants like Siri and Alexa, among other things. But deep learning systems remain vulnerable in surprising ways: stumbling when they encounter slightly unfamiliar examples in the real world or when a malicious attacker feeds it subtly-altered images. How are you and others trying to make AI more robust?
A: Until recently, AI researchers focused simply on getting machine-learning algorithms to accomplish basic tasks. Achieving even average-case performance was a major challenge. Now that performance has improved, attention has shifted to the next hurdle: improving the worst-case performance. Most of my research is focused on meeting this challenge. Specifically, I work on developing next-generation machine-learning systems that will be reliable and secure enough for mission-critical applications like self-driving cars and software that filters malicious content. We’re currently building tools to train object-recognition systems to identify what’s happening in a scene or picture, even if the images fed to the model have been manipulated. We are also studying the limits of systems that offer security and reliability guarantees. How much reliability and security can we build into machine-learning models, and what other features might we need to sacrifice to get there?
My colleague Luca Daniel, who also spoke, is working on an important aspect of this problem: developing a way to measure the resilience of a deep learning system in key situations. Decisions made by deep learning systems have major consequences, and thus it’s essential that end-users be able to measure the reliability of each of the model’s outputs. Another way to make a system more robust is during the training process. In her talk, “Robustness in GANs and in Black-box Optimization,” Stefanie Jegelka showed how the learner in a generative adversarial network, or GAN, can be made to withstand manipulations to its input, leading to much better performance.
Q: The neural networks that power deep learning seem to learn almost effortlessly: Feed them enough data and they can outperform humans at many tasks. And yet, we’ve also seen how easily they can fail, with at least three widely publicized cases of self-driving cars crashing and killing someone. AI applications in health care are not yet under the same level of scrutiny but the stakes are just as high. David Sontag focused his talk on the often life-or-death consequences when an AI system lacks robustness. What are some of the red flags when training an AI on patient medical records and other observational data?
A: This goes back to the nature of guarantees and the underlying assumptions that we build into our models. We often assume that our training datasets are representative of the real-world data we test our models on — an assumption that tends to be too optimistic. Sontag gave two examples of flawed assumptions baked into the training process that could lead an AI to give the wrong diagnosis or recommend a harmful treatment. The first focused on a massive database of patient X-rays released last year by the National Institutes of Health. The dataset was expected to bring big improvements to the automated diagnosis of lung disease until a skeptical radiologist took a closer look and found widespread errors in the scans’ diagnostic labels. An AI trained on chest scans with a lot of incorrect labels is going to have a hard time generating accurate diagnoses.
A second problem Sontag cited is the failure to correct for gaps and irregularities in the data due to system glitches or changes in how hospitals and health care providers report patient data. For example, a major disaster could limit the amount of data available for emergency room patients. If a machine-learning model failed to take that shift into account its predictions would not be very reliable.
Q: You’ve covered some of the techniques for making AI more reliable and secure. What about interpretability? What makes neural networks so hard to interpret, and how are engineers developing ways to peer beneath the hood?
A: Understanding neural-network predictions is notoriously difficult. Each prediction arises from a web of decisions made by hundreds to thousands of individual nodes. We are trying to develop new methods to make this process more transparent. In the field of computer vision one of the pioneers is Antonio Torralba, director of The Quest. In his talk, he demonstrated a new tool developed in his lab that highlights the features that a neural network is focusing on as it interprets a scene. The tool lets you identify the nodes in the network responsible for recognizing, say, a door, from a set of windows or a stand of trees. Visualizing the object-recognition process allows software developers to get a more fine-grained understanding of how the network learns.
Another way to achieve interpretability is to precisely define the properties that make the model understandable, and then train the model to find that type of solution. Tommi Jaakkola showed in his talk, “Interpretability and Functional Transparency,” that models can be trained to be linear or have other desired qualities locally while maintaining the network’s overall flexibility. Explanations are needed at different levels of resolution much as they are in interpreting physical phenomena. Of course, there’s a cost to building guarantees into machine-learning systems — this is a theme that carried through all the talks. But those guarantees are necessary and not insurmountable. The beauty of human intelligence is that while we can’t perform most tasks perfectly, as a machine might, we have the ability and flexibility to learn in a remarkable range of environments.