Boosting innovations and maximising societal impact. Role of Digital Innovation Hubs in Inspection & Maintenance robotics
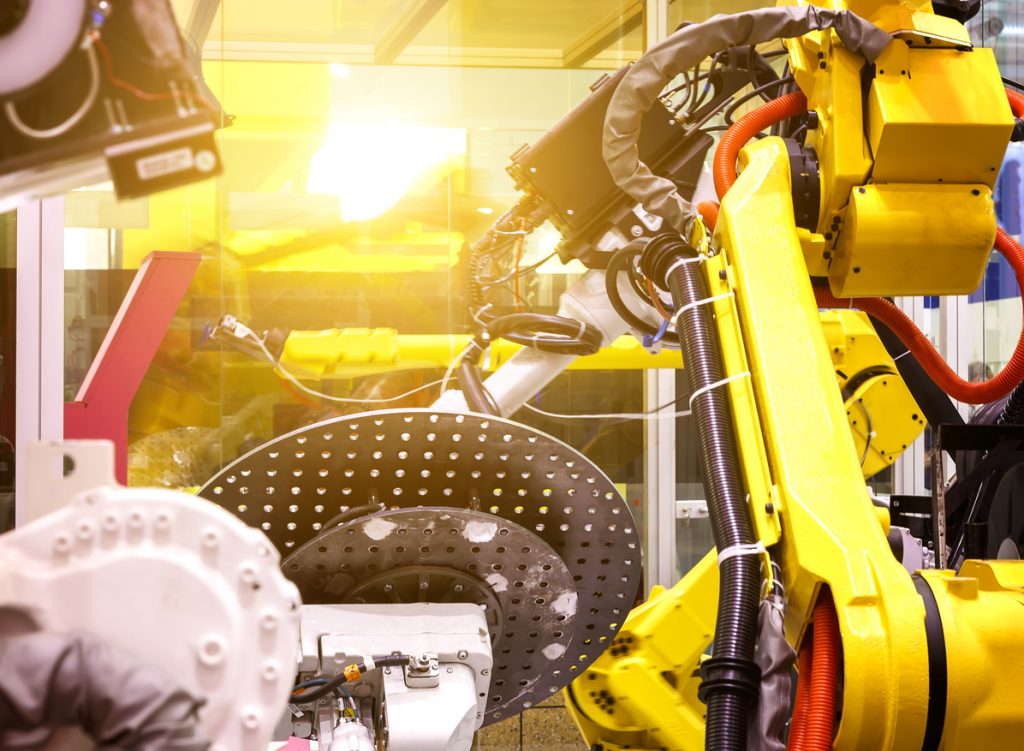
By Jovita Tautkevičiūtė
Robotics4EU is a 3-years-long EU-funded project which advocates for the wider adoption of AI-based robots in 4 sectors: healthcare, inspection and maintenance of infrastructure, agri-food, and agile production. Thus, one of the ways in which Robotics4EU raises awareness about non-technological aspects in robotics is delivering a series of workshops to involve the research community, industry representatives and citizens.
The workshop “Boosting innovations and maximising societal impact. Role of Digital Innovation Hubs (DIHs) in I&M Robotics” which took place on the 23rd of February, 2022 analysed the role and contribution of Digital Innovation Hubs (DIHs) to the widespread adoption of robotics in society. How can they enhance the implementation of robotics by SMEs and startups into their daily operations? How can they help to close the knowledge gap of non-technological issues of robotics in Inspection & Maintenance (I&M)?
These questions were analysed by five experts: Ebert von Vonderen, Ladislav Vargovcik, Maria Roca, Roi Rodriguez de Bernardo and Christophe Leroux, during the presentations and panel discussions of the workshop.
Digital Innovation Hubs
What is Digital Innovation Hub (DIH) and what role does it play? Ebert van Vonderen & Ladislav Vargovcik from DIH Robotics Hub Košice explained what DIH is and how it works with various stakeholders: universities, DIH network, SMEs. In general, DIH serves as a bridge between research organizations and industry – technology and services providers, end-users and SMEs. In the case of DIH Robotics Hub Košice, technical faculties of Košice University, Prototyping and Innovation Center established the DIH.
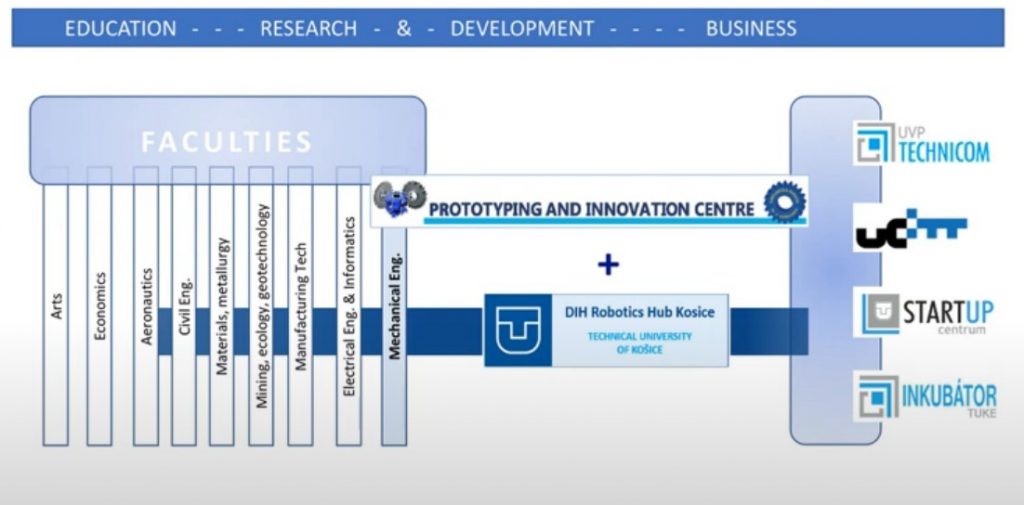
Structure of DIH Robotics Hub Košice
A DIH serves as a one-stop-shop for SMEs to bring their questions. DIHs need to ensure that SMEs have easy access to technology innovation, are provided with relevant services and training for technology adoption. Thanks to the efforts of the RIMA network, DIHs are spread over Europe and interact for I&M possibilities, competencies, and share the available opportunities across Europe.
Why are Robotics DIHs important for SMEs? They tackle many challenges that arise for companies, aiming to boost innovation, enhance and contribute to robotics implementation. Some of the challenges mentioned by Ebert van Vonderen:
- High technology levels needed, complex tasks in I&M
- High Investments needed
- Competition, profitability, ROI (profitability is in many cases a problem)
- Ecosystem maturity, differences across Europe
- Resistances from communities
- Creation of sustainable solutions
An example of DIH Robotics Hub Košice’s cooperation with INETEC, high tech company that specialises in robotics, instruments and software was presented. Magic Lancer II – a robot for nuclear inspection, was developed under the cooperation of DIH Robotics Hub Košice.
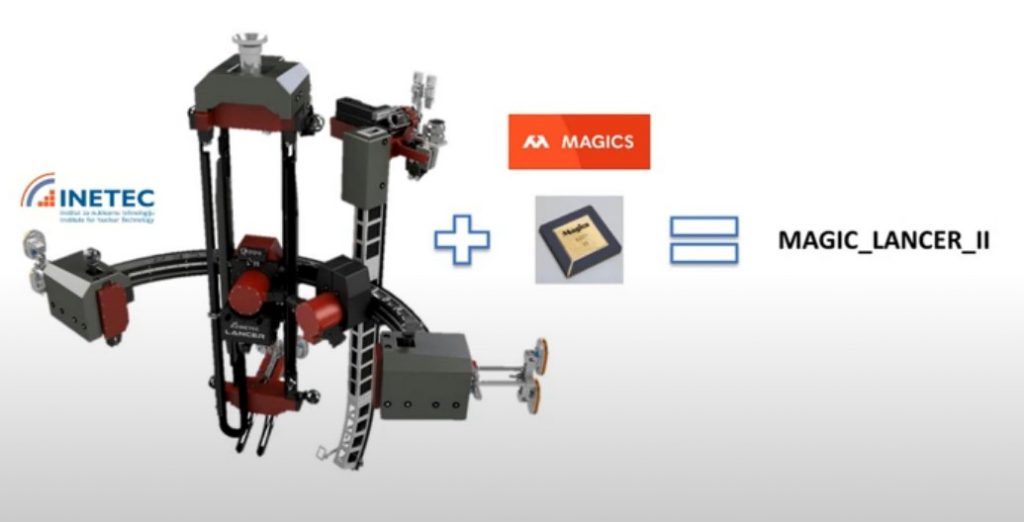
Screenshot of the Magic Lancer II presentation
How to enhance the uptake of technology?
Christophe Leroux, representing RIMA (Robotics for Inspection & Maintainance) Network explained that RIMA Network connects and inspires key stakeholders in I&M robotics and aims to accelerate innovation and uptake of robotics between these stakeholders. Its main purpose is to help digitalise European Industry. Even though the market is estimated with a huge potential for innovation (450 bn EUR/year), the bottleneck is the adoption of robotics to market – no real connection between industry and research organisations.
RIMA Network directs its efforts to establish a network of DIHs (currently, it connects 13 DIHs), focussing on robotics in I&M, fund SEMs to support experimentation, set up courses to facilitate the uptake of technologies and develop new skills, and inform people of funding opportunities for business development.
RIMA network supports SMEs by organising open calls. 15 innovative solutions were supported, with the aim to have a social-economic impact win EU with new products, services, business, established jobs, along with the reduction of costs and risks. RIMA Network provides the following services to SMEs and research organisations:
- Advising (on finances, technology)
- Proof of Concept
- Support for tech transfer
- Support for testing
- Access to Incubator’s
- Access to value chain actors
- Training and coaching
- The market study, providing information to network partners
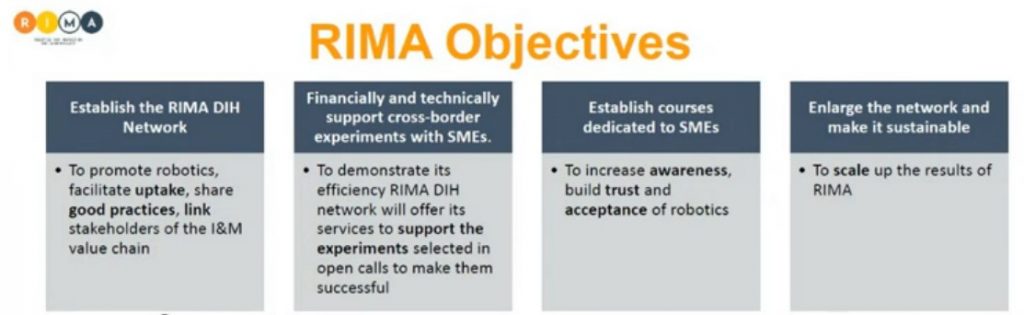
RIMA Network Objectives
ICT innovation for manufacturing SMEs
Maria Roca, I4MS Project Manager, presented I4MS (ICT Innovation for Manufacturing SMEs). It is the initiative promoted by the European Commission to foster the digital innovation of manufacturing SMEs in Europe in order to boost their competitiveness in the digital era. The I4MS goal is to contribute to the adaptation of European SMEs to the current digital transformation challenges by funding & mentorship, training and access to physical and virtual technology platforms. I4MS connects a community of 1700 members, 42% of them – SMEs. According to Maria Roca, the organisation has identified that geographical coverage of Europe is uneven and Eastern European countries need to be represented better in the network. Also, there is a gap in SMEs ’ understanding of technology advantages and network ability to consider SMEs’ interests.
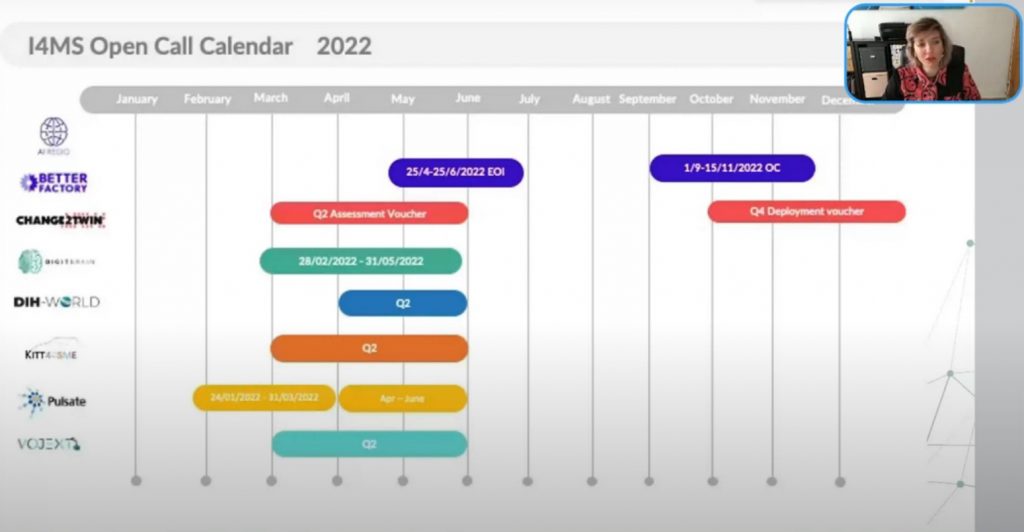
Screenshot from Maria’s Roca presentation on I4MS and Open Call Calendar
Roi Rodriguez de Bernardo, Program Manager at FundingBox presented the AI4EU platform and funding opportunities for SMEs. AI4EU is a collaborative H2020 Project that aims to:
- mobilize the entire European AI community to make AI promises real for the European Society and Economy, and
- create a leading collaborative AI European Platform to nurture economic growth.
AI4EU aims to be a catalyst between research and industry. The platform presents available funding opportunities and various tools (research, education, ethics, services).
Speaking of the funding opportunities, Roi set the scene by capturing Europe’s position in the global AI market. The AI Business market is emerging worldwide with an estimated growth of the AI market from 40,2% CAGR from 2021 to 2028. The growing focus on AI is also evident in the EU strategy. AI strategy, set by EC offers yearly 1bn EUR funding for a period of 2021-2028, expecting to leverage 20bn EUR investments. The funding is focused on SMEs adopting AI with some cases of funding for startups or technology providers developing AI solutions that could be adopted by SMEs.
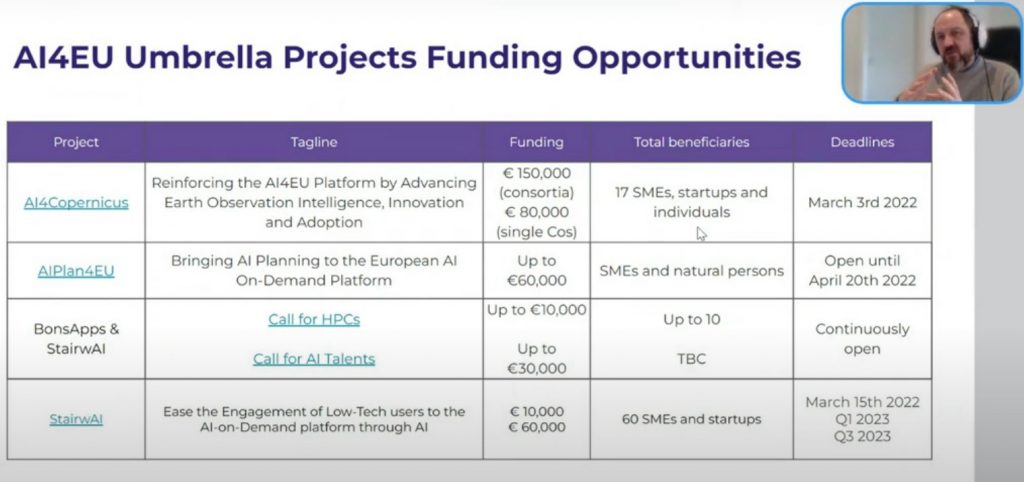
Screenshot from Roi Rodriguez de Bernardo presentation presenting Funding opportunities and open calls
Panel discussion
The discussion started with the question of whether the EU can be a powerhouse of robotics in I&M and lead the way in the race with the USA and China, also adhering to our values? It was identified that record-high investment in the industry in 2021 shows that it is possible. Even though the USA and China did not take into account the ethical aspects EU holds, they are starting to develop regulation as well. It seems that the path set by the EU is followed now by other big markets.
Considering ethical or socio-economic aspects of most importance in the field, Maria Roca identified the management of data for SMEs as a bottleneck. The difficulty lies in the hindrances to sharing data with other companies. Further, in the case of AI, ethical aspects are evaluated in all EU funded projects. From the perspective of DIH’s provided services, RIMA’s representative Christophe Leroux explained that ethical and safety aspects are taken into account in facilitated experimentation. In practice, ethical assessment is conducted for each experimentation, involving experts in the field of ethics. On a daily basis, RIMA’s work includes mentorship on ethical issues and guidelines.
The ways of enlarging the networks were also discussed. Maria Roca identified training as a main tool to attract members and showcase what they can achieve in collaboration and participation in their open calls. Networking, as a core business of DIHs, also covers the organisation of local events with experts. Also, collaboration with social scientists was emphasised as crucial in the field of AI. Annelli Rose, representative of the Robotics4EU project noted that interdisciplinary collaboration is in line with the EU priorities, where the change is foreseen to have a breeding ground.
Zooming in to the topic of robotics in I&M the social aspect of adoption was analysed. Christophe Leroux explained that there is attractiveness for robotics adoption to support certain operations because there is not much fear of losing jobs. Robots are seen as supporting their work, for example as deploying robotics in explosive, dangerous and hazardous environments. Robots are not taking away jobs, but taking away the danger. Christophe Leroux also emphasised that there is a growth in the domain of AI robotics in I&M and the main issues are related to trust and safety.
Upcoming workshop
The upcoming workshop on the 23rd of March 2022, 11-14 CET “How to make sure regulation helps and not hinders I&M robotics? Policy issues in Robotics for Inspection & Maintenance”. We will discuss how cooperation among regulators and the robotics community can be fostered and what are the most pressing legal challenges for the I&M application area of robotics. We will be investigating how to ensure the accessibility of objective information and enhance the capacity of regulators to comprehend technical aspects, risks and opportunities of robotics in I&M. We hope to leave the workshop with industry insights and specific areas for improvement for the future, which as a project we will continue to explore in our activities. Register here.
BirdBot is energy-efficient thanks to nature as a model
Safe and powerful robotics for near-human tasks
From remote tourism to metaverse, a new robotic avatar made in Italy
Computer simulation brings us closer to schools of fishlike underwater research drones
Robotic telekinesis: Allowing humans to remotely operate and train robotic hands
Geek+ Technology Upgrades World’s Largest Smart LED Manufacturing Facility
Seamless transitions between autonomous robot capabilities and human intervention in construction robotics
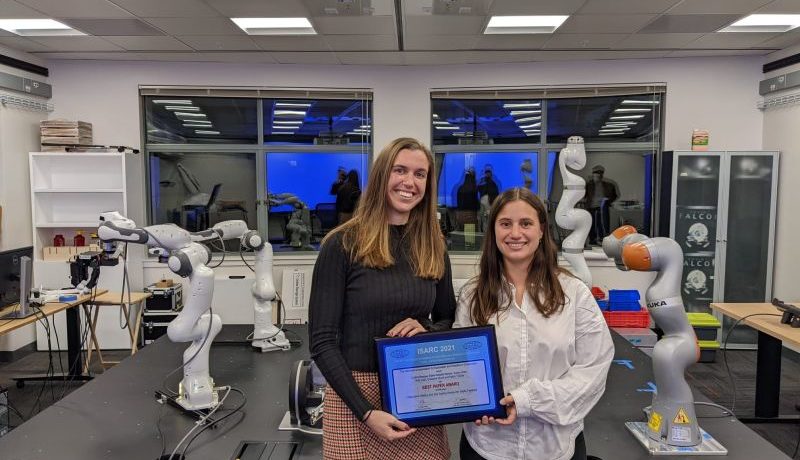
Congratulations to the winners of the best paper award of the International Association for Automation and Robotics in Construction 2021. The team around Cynthia Brosque, Elena Galbally, Prof. Martin Fischer, and Prof. Oussama Khatib did excellent groundwork for construction robotics. With permission, Silicon Valley Robotics is reposting the first parts of the paper below. It is also available on the IAARC website.
GOLDBECK US Inc. helped define construction robotics use-cases that generate real-world value on their job sites. In the first phase of the project, they collected data to understand the tasks on site holistically. Subsequently, simulations on how robots perform the job were created. Using this information, the researchers of Stanford University | CIFE – Center for Integrated Facility Engineering then developed suitable robotic prototypes. GOLDBECK supplied physical building components to test the prototypes under realistic conditions.
GOLDBECK is now hunting for robotics companies to help us transition this research into a real-world robotic application!
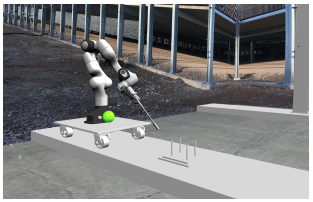
Abstract
Due to their unstructured and dynamic nature, construction sites present many challenges for robotic automation of tasks. Integrating human-robot collaboration (HRC) is critical for task success and implementation feasibility. This is particularly important for contact-rich tasks and other complex scenarios which require a level of reasoning that cannot be accomplished by a fully autonomous robot. Currently, many solutions rely on precise teleoperation that requires one operator per robot. Alternatively, one operator may oversee several semi-autonomous robots. However, the operators do not have the sensory feedback needed to adequately leverage their expertise and craftsmanship. Haptic interfaces allow for intuitive human-robot collaboration by providing rich contact feedback. This paper presents two human-robot collaboration solutions for welding and joint sealing through the use of a haptic device. Our approach allows for seamless transitions between autonomous robot capabilities and human intervention with rich contact feedback. Additionally, this work opens the door to intuitive programming of new tasks through haptic human demonstration.
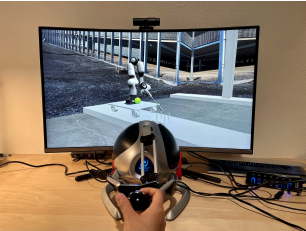
1 Introduction
In recent years, progress in mobility, manipulation skills, and AI reasoning have started to enable the use of robots in space, underwater, homes, agriculture, and construction [1]. A particularly important area of interest is the automation of dangerous, strenuous, and laborintensive tasks [2].
Construction sites are especially challenging environments for autonomous robots because of their highly unpredictable and unstructured nature [3, 4]. Hence, fully autonomous robots that replace human labor are not the most feasible or ideal solution. The majority of current approaches rely on a human operator that oversees a single task autonomous robot. The operator receives only visual feedback and is limited in the type of input and recovery from failure he can provide due to the lack of an intuitive interface to do so. [4] attributed this lack in technical flexibility of construction robots to the fact that early construction solutions imitated systems initially developed for industrial fabrication [5].
Some tasks are structured enough to be autonomously performed by a robot with little human input, but many require a more flexible approach that incorporates a higher degree of human reasoning and intuition [6]. Given this reality, a method to design construction robots should be flexible enough to allow varying levels of human-robot collaboration depending on the task.
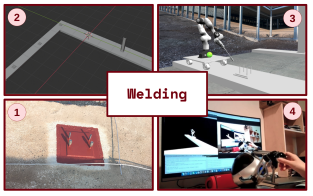
Haptic devices (Fig.1) provide an effective interface for collaboration by allowing the human to (1) feel the contact forces between the robot’s end-effector and the environment [7], and (2) easily intervene and control the robot motion in scenarios that the autonomous behaviors are not able to handle successfully [8]. Additionally, data from these haptic interventions can be collected and used to learn new autonomous skills. Remote robot control using a haptic interface has been tested in fields such as surgery [9] and underwater exploration [1], but has not yet been widely implemented in construction.
In previous work, the authors explored human-robot collaboration solutions to five hazardous and repetitive construction tasks: installing drywall, painting, bolting, welding, and sealing precast concrete slab joints [10]. Our industry partner, Goldbeck, was interested in automating these assembly and finishing tasks that require on-site, repetitive manual effort, ergonomically challenging positions, and working from dangerous heights. [10] outlines a method for designing collaborative robotic solutions with haptic feedback and to assess their feasibility in simulation.
In this paper, we focus on two of the previously explored tasks (steel welding and sealing precast concrete joints) and apply the aforementioned method to design more flexible collaborative solutions. Different from [10], we propose relying primarily on the robot’s functional autonomy and using haptics as an effective and intuitive way to intervene in unexplored or failure scenarios. Force data from the recovery strategy employed by the operator can be recorded and used to learn from demonstration and augment the robot’s autonomy. Over time, the robot will require less human intervention. This higher degree of autonomy could allow a single operator to supervise many robots at once, overcoming the problems of teleoperation in which one operator per robot is needed.
2 Related Work
While factories have typically separated workers from robots due to safety concerns, human-robot collaboration cannot be overlooked in construction, as robots and humans share one workspace [2]. This requires devising solutions that allow us to effectively combine the workers’ expertise with the robots’ autonomous skills.
Construction literature has studied the use of teleoperation devices [11, 12, 7], particularly focusing on construction machinery, such as excavators. These solutions often involve cameras for visual feedback and GPS sensors for navigation, which can be sufficient to accomplish low dexterity tasks with increased operator safety. However, [7] states that complex tasks involving contact greatly benefit from additional sensory feedback such as tactile information. Furthermore, teleoperation solutions rely heavily on the operator’s guidance and do not fully exploit the autonomous capabilities of the robot.
A different set of collaborative solutions currently used onsite have semi-autonomous robots with a human supervisor that oversees the tasks such as drywall installation, concrete drilling, and layout [13]. The supervisor can provide simple inputs to the robot, such as when to start or stop the operation, while the robot handles the rest of the task. This approach makes better use of modern robotics capabilities and allows a single operator to manage several robots. However, the interfaces used to provide inputs to the robot are often too simplistic to allow recovery from failure.
In the event of a robot failure during task execution, joysticks and control pendants do not always provide enough feedback for the operator to intervene in an effective way that enables timely task completion. Additionally, there is currently no streamlined way to learn from the operator’s intervention and use this data to improve the robot’s autonomous capabilities.
By allowing the operator to feel the contact between the robot and its environment, haptic devices increase the range of scenarios in which the operator can aid in failure recovery [14]. Additionally, we can easily record both force and position data during the operator’s intervention. These human demonstrations of recovery strategies can allow the robot to learn new skills [15] and augment its functional autonomy.
Haptic devices have been used by the construction industry in combination with virtual reality for task training purposes [16]. The technology has allowed workers to train in a safe environment with realistic task conditions. However, haptics are still a novel technology in construction applications and field use has not been reported.
Current algorithms for haptic control of robots [17] can handle large communication delays, making them effective interfaces for remote intervention at long distances. In [1] an operator haptically controls an underwater ocean exploration robot from a distance of 100m.
Finally, [18] provides an example that integrates two modalities of robot control: autonomous robot behavior and expert human-guided motion interactions. In this study, a group of mobile robot arms successfully installed drywall boards in simulation with flexible human intervention.
This body of prior work illustrates how keeping the human in the loop with adequate feedback can facilitate successful task automation in complex, unstructured environments. Moreover, it highlights the value of haptics as a way to provide a flexible and effective interface for human-robot collaboration as well as teaching robots new autonomous skills.