Setting Yourself Up for Future Robotics Industry Success
Sensors set to revolutionise brain-controlled robotics
Robot density nearly doubled globally
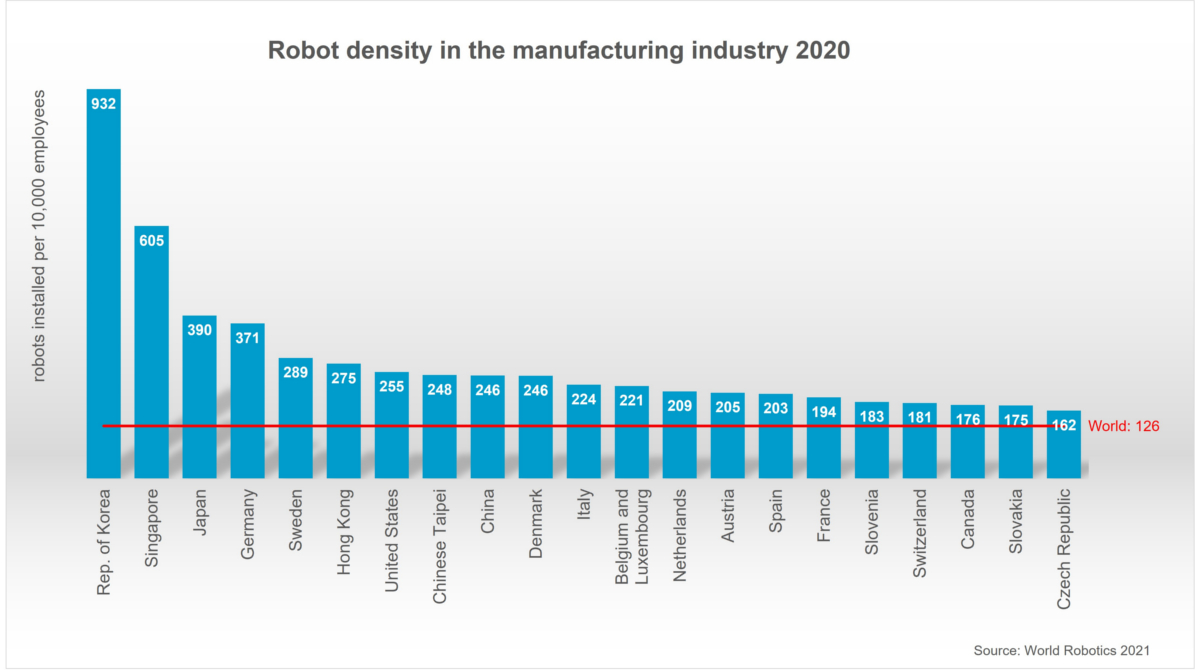
The use of industrial robots in factories around the world is accelerating at a high rate: 126 robots per 10,000 employees is the new average of global robot density in the manufacturing industries – nearly double the number five years ago (2015: 66 units). This is according to the 2021 World Robot Report.
By regions, the average robot density in Asia/Australia is 134 units, in Europe 123 units and in the Americas 111 units. The top 5 most automated countries in the world are: South Korea, Singapore, Japan, Germany, and Sweden.
“Robot density is the barometer to track the degree of automation adoption in the manufacturing industry around the world,” says Milton Guerry, President of the International Federation of Robotics.
Asia
The development of robot density in China is the most dynamic worldwide: Due to the significant growth of robot installations, the density rate rose from 49 units in 2015 to 246 units in 2020. Today, China’s robot density ranks 9th globally compared to 25th just five years ago.
Asia is also the home of the country with the world´s highest robot density in the manufacturing industry: the Republic of Korea has held this position since 2010. The country’s robot density exceeds the global average seven-fold (932 units per 10,000 workers). Robot density had been increasing by 10% on average each year since 2015. With its globally recognized electronics industry and a distinct automotive industry, the Korean economy is based on the two largest areas for industrial robots.
Singapore takes second place with a rate of 605 robots per 10,000 employees in 2020. Singapore’s robot density had been growing by 27% on average each year since 2015.
Japan ranked third in the world: In 2020, 390 robots were installed per 10,000 employees in the manufacturing industry. Japan is the world´s predominant industrial robot manufacturer: The production capacity of Japanese suppliers reached 174,000 units in 2020. Today, Japan´s manufacturers deliver 45% of the global robot supply.
North America
Robot density in the United States rose from 176 units in 2015 to 255 units in 2020. The country ranks seventh in the world – ahead of Chinese Taipei (248 units) and China (246 units). The modernization of domestic production facilities has boosted robot sales in the United States. The use of industrial robots also aids to achieve decarbonization targets e.g. in the cost-efficient production of solar panels and in the continued transition towards electric vehicles. Several car manufacturers have announced investments to further equip their factories for new electric drive car models or to increase capacity for battery production. These major projects will create demand for industrial robots in the next few years.
Europe
Europe´s most automated country is Germany – ranking 4th worldwide with 371 units. The annual supply had a share of 33% of total robot sales in Europe 2020 – 38% of Europe’s operational stock is in Germany. The German robotics industry is recovering, mainly driven by strong overseas business rather than by the domestic or European market. Robot demand in Germany is expected to grow slowly, mainly supported by demand for low-cost robots in the general industries and outside traditional manufacturing.
France has a robot density of 194 units (ranking 16th in the world), which is well above the global average of 126 robots and relatively similar compared to other EU countries like Spain (203 units), Austria (205 units) or The Netherlands (209 units). EU members like Sweden (289 units), Denmark (246 units) or Italy (224 units), have a significantly higher degree of automation in the manufacturing segment.
As the only G7 country – the UK has a robot density below the world average of 126 units with 101 units, ranking 24th. Five years ago, the UK´s robot density was 71 units. The exodus of foreign labor after Brexit increased the demand for robots in 2020. This situation is expected to prevail in near future, the modernization of the UK manufacturing industry will also be boosted by massive tax incentives, the “super-deduction”: From April 2021 until March 2023, companies can claim 130% of capital allowances as a tax relief for plant and machinery investments.
Call for robot holiday videos 2021
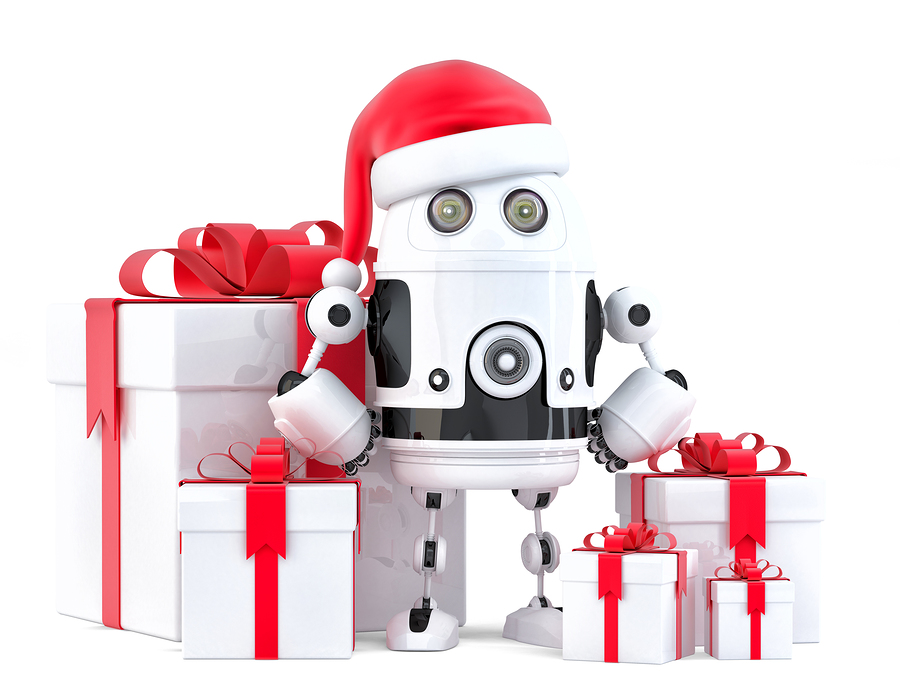
That’s right! You better not run, you better not hide, you better watch out for brand new robot holiday videos on Robohub!
Drop your submissions down our chimney at daniel.carrillozapata@robohub.org and share the spirit of the season.
For inspiration, here are our two first videos:
New haptic device communicates emotion with nearly 80% accuracy of human touch
You Know Your Company Needs Robotics, but Don’t Know Where to Start. Here’s How.
A new micro aerial robot based on dielectric elastomer actuators
Heliogen Demonstrates AI-Powered Autonomous Robot Designed to Lower Installation and Maintenance Costs of Full-Scale Concentrated Solar Plants
Giving bug-like bots a boost
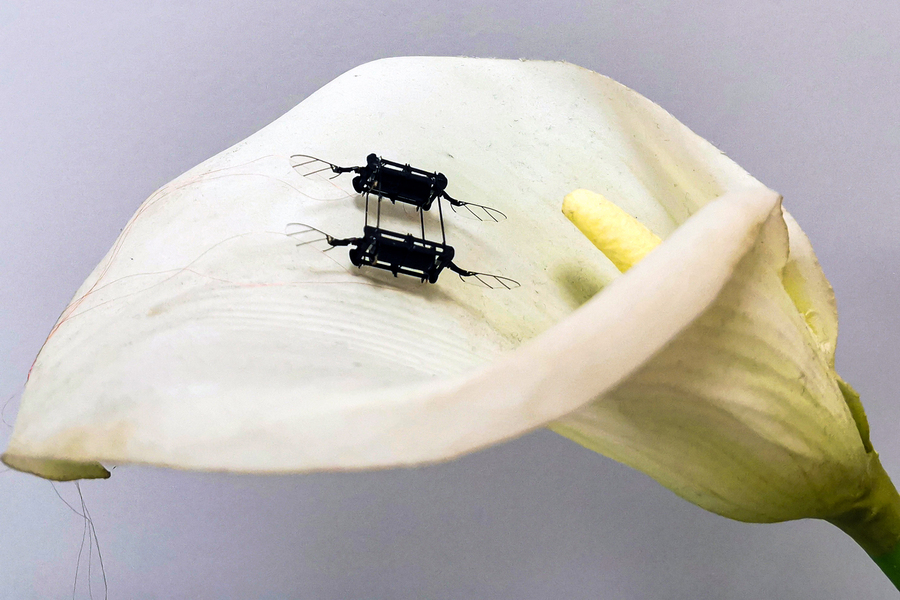
MIT researchers have pioneered a new fabrication technique that enables them to produce low-voltage, power-dense, high endurance soft actuators for an aerial microrobot. Credits: Courtesy of the researchers
By Adam Zewe | MIT News Office
When it comes to robots, bigger isn’t always better. Someday, a swarm of insect-sized robots might pollinate a field of crops or search for survivors amid the rubble of a collapsed building.
MIT researchers have demonstrated diminutive drones that can zip around with bug-like agility and resilience, which could eventually perform these tasks. The soft actuators that propel these microrobots are very durable, but they require much higher voltages than similarly-sized rigid actuators. The featherweight robots can’t carry the necessary power electronics that would allow them fly on their own.
Now, these researchers have pioneered a fabrication technique that enables them to build soft actuators that operate with 75 percent lower voltage than current versions while carrying 80 percent more payload. These soft actuators are like artificial muscles that rapidly flap the robot’s wings.
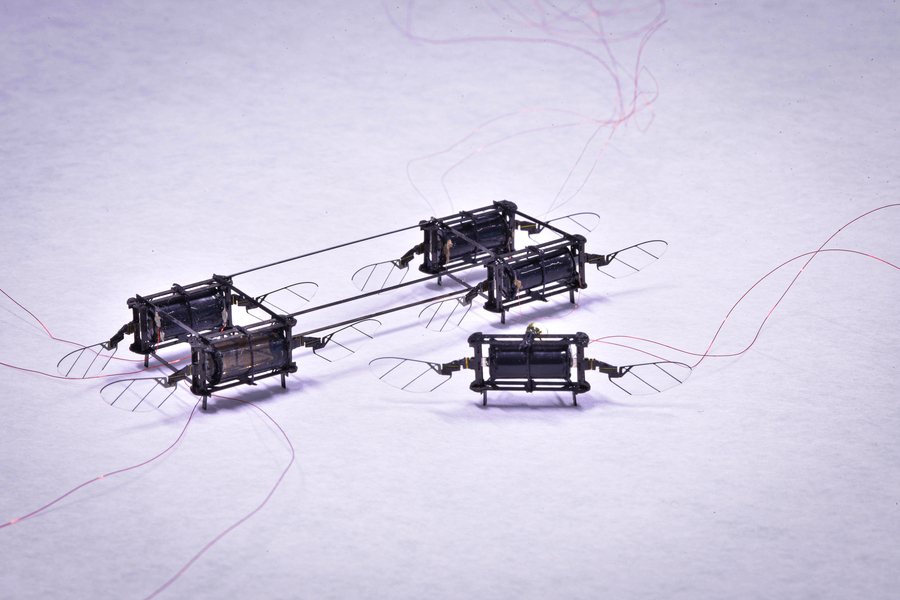
The artificial muscles vastly improve the robot’s payload and allow it to achieve best-in-class hovering performance. Image: Kevin Chen
This new fabrication technique produces artificial muscles with fewer defects, which dramatically extends the lifespan of the components and increases the robot’s performance and payload.
“This opens up a lot of opportunity in the future for us to transition to putting power electronics on the microrobot. People tend to think that soft robots are not as capable as rigid robots. We demonstrate that this robot, weighing less than a gram, flies for the longest time with the smallest error during a hovering flight. The take-home message is that soft robots can exceed the performance of rigid robots,” says Kevin Chen, who is the D. Reid Weedon, Jr. ’41 assistant professor in the Department of Electrical Engineering and Computer Science, the head of the Soft and Micro Robotics Laboratory in the Research Laboratory of Electronics (RLE), and the senior author of the paper.
Chen’s coauthors include Zhijian Ren and Suhan Kim, co-lead authors and EECS graduate students; Xiang Ji, a research scientist in EECS; Weikun Zhu, a chemical engineering graduate student; Farnaz Niroui, an assistant professor in EECS; and Jing Kong, a professor in EECS and principal investigator in RLE. The research has been accepted for publication in Advanced Materials and is included in the jounal’s Rising Stars series, which recognizes outstanding works from early-career researchers.
Making muscles
The rectangular microrobot, which weighs less than one-fourth of a penny, has four sets of wings that are each driven by a soft actuator. These muscle-like actuators are made from layers of elastomer that are sandwiched between two very thin electrodes and then rolled into a squishy cylinder. When voltage is applied to the actuator, the electrodes squeeze the elastomer, and that mechanical strain is used to flap the wing.
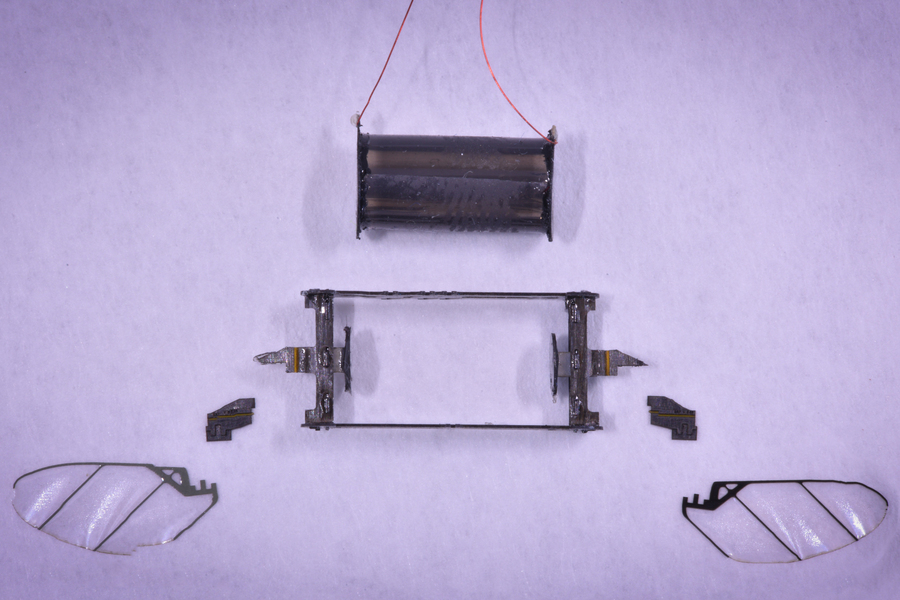
The rectangular microrobot, which weighs less than one-fourth of a penny, has four sets of wings that are each driven by a soft actuator. Credits: Courtesy of the researchers
The more surface area the actuator has, the less voltage is required. So, Chen and his team build these artificial muscles by alternating between as many ultrathin layers of elastomer and electrode as they can. As elastomer layers get thinner, they become more unstable.
For the first time, the researchers were able to create an actuator with 20 layers, each of which is 10 micrometers in thickness (about the diameter of a red blood cell). But they had to reinvent parts of the fabrication process to get there.
One major roadblock came from the spin coating process. During spin coating, an elastomer is poured onto a flat surface and rapidly rotated, and the centrifugal force pulls the film outward to make it thinner.
“In this process, air comes back into the elastomer and creates a lot of microscopic air bubbles. The diameter of these air bubbles is barely 1 micrometer, so previously we just sort of ignored them. But when you get thinner and thinner layers, the effect of the air bubbles becomes stronger and stronger. That is traditionally why people haven’t been able to make these very thin layers,” Chen explains.
He and his collaborators found that if they perform a vacuuming process immediately after spin coating, while the elastomer was still wet, it removes the air bubbles. Then, they bake the elastomer to dry it.
Removing these defects increases the power output of the actuator by more than 300 percent and significantly improves its lifespan, Chen says.
The researchers also optimized the thin electrodes, which are composed of carbon nanotubes, super-strong rolls of carbon that are about 1/50,000 the diameter of human hair. Higher concentrations of carbon nanotubes increase the actuator’s power output and reduce voltage, but dense layers also contain more defects.
For instance, the carbon nanotubes have sharp ends and can pierce the elastomer, which causes the device to short out, Chen explains. After much trial and error, the researchers found the optimal concentration.
Another problem comes from the curing stage — as more layers are added, the actuator takes longer and longer to dry.
“The first time I asked my student to make a multilayer actuator, once he got to 12 layers, he had to wait two days for it to cure. That is totally not sustainable, especially if you want to scale up to more layers,” Chen says.
They found that baking each layer for a few minutes immediately after the carbon nanotubes are transferred to the elastomer cuts down the curing time as more layers are added.
Best-in-class performance
After using this technique to create a 20-layer artificial muscle, they tested it against their previous six-layer version and state-of-the-art, rigid actuators.
During liftoff experiments, the 20-layer actuator, which requires less than 500 volts to operate, exerted enough power to give the robot a lift-to-weight ratio of 3.7 to 1, so it could carry items that are nearly three times its weight.
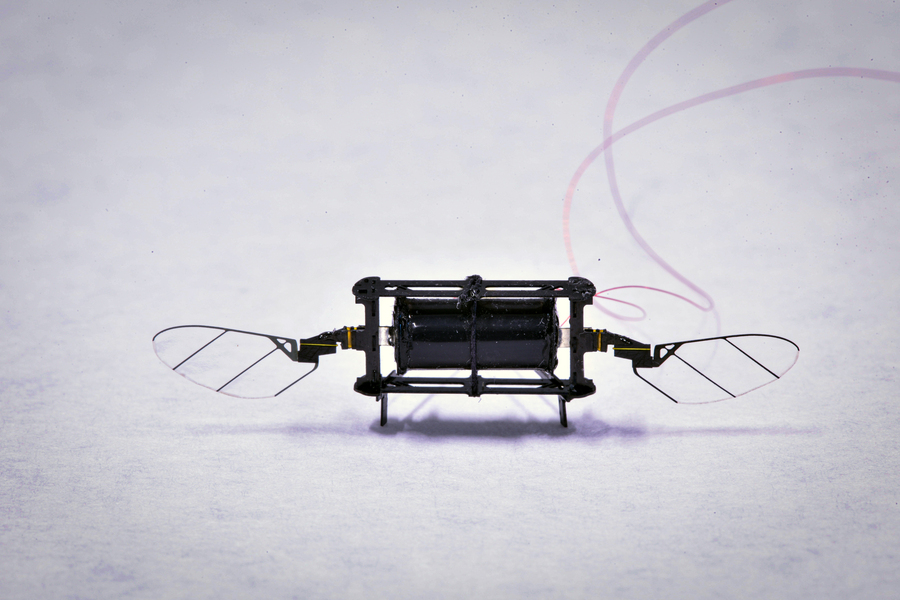
“We demonstrate that this robot, weighing less than a gram, flies for the longest time with the smallest error during a hovering flight,” says Kevin Chen. Credits: Courtesy of the researchers
They also demonstrated a 20-second hovering flight, which Chen says is the longest ever recorded by a sub-gram robot. Their hovering robot held its position more stably than any of the others. The 20-layer actuator was still working smoothly after being driven for more than 2 million cycles, far outpacing the lifespan of other actuators.
“Two years ago, we created the most power-dense actuator and it could barely fly. We started to wonder, can soft robots ever compete with rigid robots? We observed one defect after another, so we kept working and we solved one fabrication problem after another, and now the soft actuator’s performance is catching up. They are even a little bit better than the state-of-the-art rigid ones. And there are still a number of fabrication processes in material science that we don’t understand. So, I am very excited to continue to reduce actuation voltage,” he says.
Chen looks forward to collaborating with Niroui to build actuators in a clean room at MIT.nano and leverage nanofabrication techniques. Now, his team is limited to how thin they can make the layers due to dust in the air and a maximum spin coating speed. Working in a clean room eliminates this problem and would allow them to use methods, such as doctor blading, that are more precise than spin coating.
While Chen is thrilled about producing 10-micrometer actuator layers, his hope is to reduce the thickness to only 1 micrometer, which would open the door to many applications for these insect-sized robots.
This work is supported, in part, by the MIT Research Laboratory of Electronics and a Mathworks Graduate Fellowship.
Unconventional Space Robots
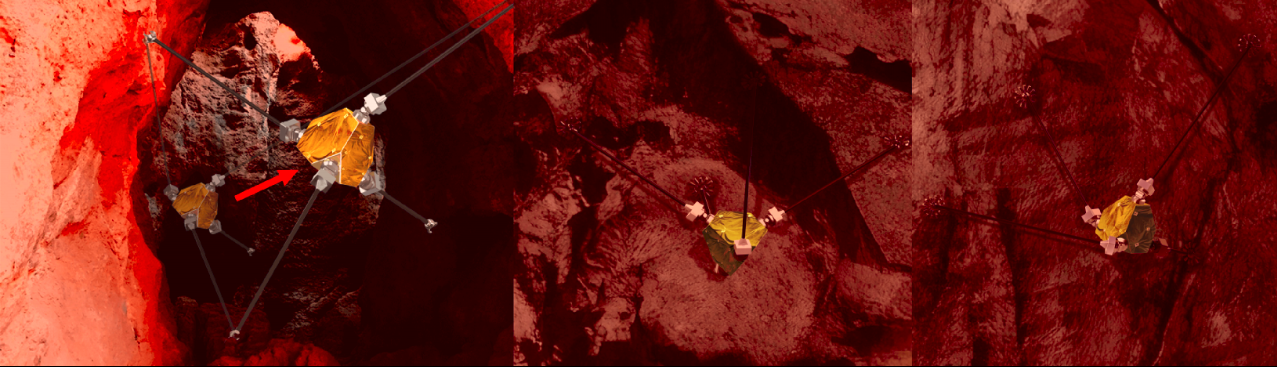
Credits: Marco Pavone
Lilly interviews Stephanie Schneider, a PhD candidate at Stanford working on unconventional space robotics. Schneider explains her work on Reachbot, a long-reach crawling and anchoring robot, which repurposes extendable booms for mobile manipulation. They discuss the challenges and exciting elements of robotic prototyping for low-gravity or otherwise unique environments.
https://youtu.be/0RqFgOHqz7E
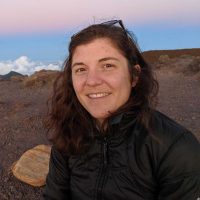
Stephanie Schneider
Stephanie Schneider is a Ph.D. candidate in Aeronautics and Astronautics in the Autonomous Systems Laboratory at Stanford, working with Professor Marco Pavone. She received her BS in Mechanical Engineering from Cornell University in 2014. Stephanie’s research interests include real-time spacecraft motion-planning, grasping and manipulation in space, and adaptive control for autonomous robotics.
Links
- Download mp3 (41.7 MB)
- Subscribe to Robohub using iTunes, RSS, or Spotify
- Support us on Patreon