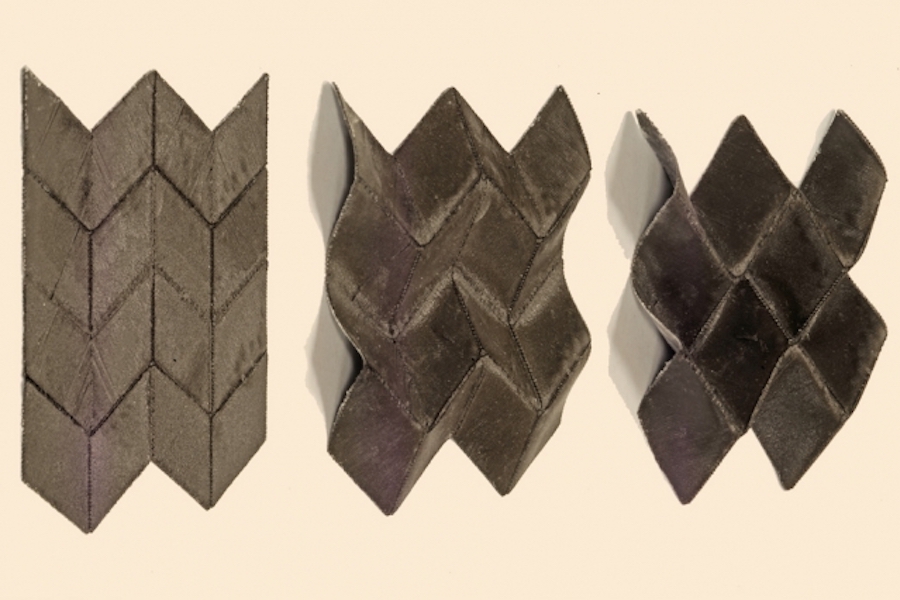
Photo: Felice Frankel
By Jennifer Chu
MIT engineers have created soft, 3-D-printed structures whose movements can be controlled with a wave of a magnet, much like marionettes without the strings.
The menagerie of structures that can be magnetically manipulated includes a smooth ring that wrinkles up, a long tube that squeezes shut, a sheet that folds itself, and a spider-like “grabber” that can crawl, roll, jump, and snap together fast enough to catch a passing ball. It can even be directed to wrap itself around a small pill and carry it across a table.
The researchers fabricated each structure from a new type of 3-D-printable ink that they infused with tiny magnetic particles. They fitted an electromagnet around the nozzle of a 3-D printer, which caused the magnetic particles to swing into a single orientation as the ink was fed through the nozzle. By controlling the magnetic orientation of individual sections in the structure, the researchers can produce structures and devices that can almost instantaneously shift into intricate formations, and even move about, as the various sections respond to an external magnetic field.
Xuanhe Zhao, the Noyce Career Development Professor in MIT’s Department of Mechanical Engineering and Department of Civil and Environmental Engineering, says the group’s technique may be used to fabricate magnetically controlled biomedical devices.
“We think in biomedicine this technique will find promising applications,” Zhao says. “For example, we could put a structure around a blood vessel to control the pumping of blood, or use a magnet to guide a device through the GI tract to take images, extract tissue samples, clear a blockage, or deliver certain drugs to a specific location. You can design, simulate, and then just print to achieve various functions.”
Zhao and his colleagues have published their results today in the journal Nature. His co-authors include Yoonho Kim, Hyunwoo Yuk, and Ruike Zhao of MIT, and Shawn Chester of the New Jersey Institute of Technology.
A shifting field
The team’s magnetically activated structures fall under the general category of soft actuated devices — squishy, moldable materials that are designed to shape-shift or move about through a variety of mechanical means. For instance, hydrogel devices swell when temperature or pH changes; shape-memory polymers and liquid crystal elastomers deform with sufficient stimuli such as heat or light; pneumatic and hydraulic devices can be actuated by air or water pumped into them; and dielectric elastomers stretch under electric voltages.
But hydrogels, shape-memory polymers, and liquid crystal elastomers are slow to respond, and change shape over the course of minutes to hours. Air- and water-driven devices require tubes that connect them to pumps, making them inefficient for remotely controlled applications. Dielectric elastomers require high voltages, usually above a thousand volts.
“There is no ideal candidate for a soft robot that can perform in an enclosed space like a human body, where you’d want to carry out certain tasks untethered,” Kim says. “That’s why we think there’s great promise in this idea of magnetic actuation, because it is fast, forceful, body-benign, and can be remotely controlled.”
Other groups have fabricated magnetically activated materials, though the movements they have achieved have been relatively simple. For the most part, researchers mix a polymer solution with magnetic beads, and pour the mixture into a mold. Once the material cures, they apply a magnetic field to uniformly magnetize the beads, before removing the structure from the mold.
“People have only made structures that elongate, shrink, or bend,” Yuk says. “The challenge is, how do you design a structure or robot that can perform much more complicated tasks?”
Domain game
Instead of making structures with magnetic particles of the same, uniform orientation, the team looked for ways to create magnetic “domains” — individual sections of a structure, each with a distinct orientation of magnetic particles. When exposed to an external magnetic field, each section should move in a distinct way, depending on the direction its particles move in response to the magnetic field. In this way, the group surmised that structures should carry out more complex articulations and movements.
With their new 3-D-printing platform, the researchers can print sections, or domains, of a structure, and tune the orientation of magnetic particles in a particular domain by changing the direction of the electromagnet encircling the printer’s nozzle, as the domain is printed.
The team also developed a physical model that predicts how a printed structure will deform under a magnetic field. Given the elasticity of the printed material, the pattern of domains in a structure, and the way in which an external magnetic field is applied, the model can predict the way an overall structure will deform or move. Ruike found that the model’s predictions closely matched with experiments the team carried out with a number of different printed structures.
In addition to a rippling ring, a self-squeezing tube, and a spider-like grabber, the team printed other complex structures, such as a set of “auxetic” structures that rapidly shrink or expand along two directions. Zhao and his colleagues also printed a ring embedded with electrical circuits and red and green LED lights. Depending on the orientation of an external magnetic field, the ring deforms to light up either red or green, in a programmed manner.
“We have developed a printing platform and a predictive model for others to use. People can design their own structures and domain patterns, validate them with the model, and print them to actuate various functions,” Zhao says. “By programming complex information of structure, domain, and magnetic field, one can even print intelligent machines such as robots.”
Jerry Qi, professor of mechanical engineering at Georgia Tech, says the group’s design can enable a range of fast, remotely controlled soft robotics, particularly in the biomedical field.
“This work is very novel,” says Qi, who was not involved in the research. “One could use a soft robot inside a human body or somewhere that is not easily accessible. With this technology reported in this paper, one can apply a magnetic field outside the human body, without using any wiring. Because of its fast responsive speed, the soft robot can fulfill many actions in a short time. These are important for practical applications.”
This research was supported, in part, by the National Science Foundation, the Office of Naval Research, and the MIT Institute for Soldier Nanotechnologies.